News

A sustainable chemical industry
In Aachen, Germany, the Werner Siemens Foundation’s “ project of the century” is taking shape: the multidisciplinary team at “catalaix”, the new WSS Research Centre, are developing catalysis-driven methods to enable a multidimensional circular economy in the chemical industry—in particular for the mixed plastic sector. The researchers have already attained the first positive results for various kinds of plastics, as a recent visit to the lab has revealed.
From the outside, the Institute of Technical and Macromolecular Chemistry (ITMC) at RWTH Aachen University makes none-too modern an impression. But sometimes looks deceive: although the building dates back to the 1970s, the labs it houses are state of the art. And on the inside, researchers are doing no less than constructing the chemistry industry of tomorrow.
The ITMC facilities are the nerve centre of “catalaix: Catalysis for a circular economy”, the Werner Siemens Foundation’s project of the century and winner of the high-calibre ideas competition the Foundation held to mark its centennial anniversary. Endowed with a ten-year grant of one hundred million Swiss francs, catalaix is led by Regina Palkovits, Chair of Heterogeneous Catalysis and Technical Chemistry, and Jürgen Klankermayer, Chair of Translational Molecular Catalysis at RWTH. Now, the Aachen team are setting up a research centre that has the ambitious goal of enabling a recycling-based chemical industry.
Put very simply, today’s chemical industry uses ingenious, technically complex procedures to transform petroleum-based raw materials into a wide range of products. “These are products with maximum functionality and high economic viability,” Regina Palkovits says. “Until now, however, little thought has been given to what happens after they reach the end of their life cycle.” As a result, most chemically manufactured goods are thrown away as waste. “Society can no longer afford to do this,” Palkovits continues. “It’s critical that we move away from a linear economy and towards a circular economy.”
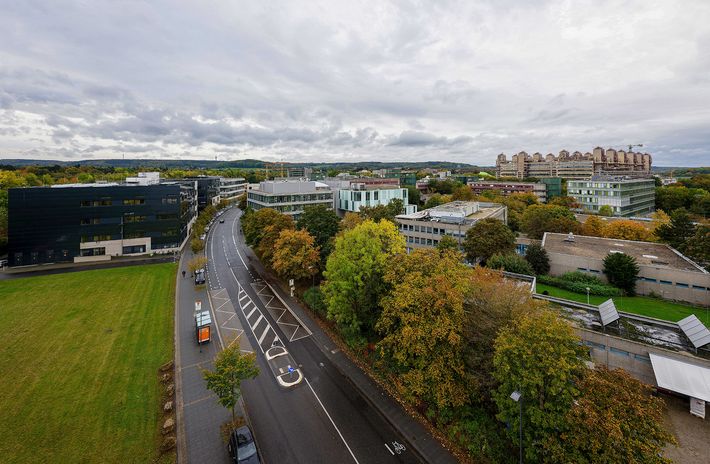
Mountains of plastic waste
The first research priority of the catalaix team is the plastics sector, which provides a prime example of both the advantages and the problems inherent in today’s chemical industry. Modern life would be unthinkable without plastics: we find them in packaging, pipes, flooring, insulation, car dashboards, tyres, medical products, cosmetics, upholstery and textile fibres. Indeed, there’s hardly an industrial sector that does without plastic. And with 400 million tonnes of new plastics produced every year, by mid-century, the quantity churned out since 1950 will come to some sixteen gigatons, as much as the combined weight of all the animals and fungi on earth.
This onslaught of plastic causes two main problems: first, its manufacture consumes vast amounts of energy and second, it generates massive mountains of waste. “A volume equal to fifty kilograms of plastic per capita is fabricated every year, and for every citizen in industrialised countries like Germany, the plastics industry consumes 1.2 tonnes of crude oil—these are enormous figures,” says Jürgen Klankermayer. In 2050, it’s estimated that emissions from the plastics sector will equal those caused by 800 coal-fired power plants. And in the same time frame, the floating islands of plastic waste in the world’s oceans could mushroom to the size of France.
Today, only nine percent of all plastics are recycled; examples include PET bottles that are shredded and remade as new PET bottles. However, one-dimensional cycles like this are unsuitable for a holistic, multidimensional approach, mainly because plastics belong to a highly complex, heterogeneous class of materials with very different chemical structures. Indeed, there are more than 200 different plastic classes on the market. In addition, various quantities are manufactured, hundreds of additives are used and the products’ lifespans differ: while packaging film may have done its job within a few weeks, building insulation is expected to last thirty years.
Modular recycling
The core concept in catalaix is to develop a type of modular principle for recycling these diverse materials: the idea is to break down the various kinds of plastic waste into molecular building blocks that are so versatile that they can be utilised for making a wide range of very different new materials—all according to demand. “This way, previously isolated cycles of matter can be linked together and a flexible circular economy can be created,” Jürgen Klankermayer says.
Regina Palkovits adds that it’s vital to look at waste materials as valuable resources. “A considerable amount of energy and valuable synthesis reactions are stored in plastic materials; it’s not wise to throw them away or burn them.” That’s why the researchers want to instrumentalise recycling technologies to produce molecular building blocks that retain the greatest possible chemical value. At the same time, they want to make these future building blocks more sustainable, ideally even biodegradable, especially when transport routes are long or when the carbon footprint caused by collection would be unjustifiable.
Catalysis—the process that accelerates the speed of chemical reactions or makes them possible in the first place—is at the heart of catalaix. “Up to now, chemists have mainly sought new catalysts to create bonds,” Jürgen Klankermayer explains. “In our work, we’re seeking catalysts to selectively break bonds.”
Beyond plastics
The capacity to break existing bonds is also important for the goal of developing new chemical products that are sustainable and easier to recycle than today’s plastics. “For these new products, it’s critical to think about recycling from the outset,” Palkovits says. One idea is to insert a type of predetermined breaking point into newly developed molecules to make it easy for a catalyst to act on the bond at the end of a product’s life cycle. “These methods can also be applied on other materials, not just plastics,” Palkovits says. “That’s why catalaix shouldn’t be seen as only a plastics project. It will have much broader implications for driving the chemical industry towards sustainability.”
Indeed, the general idea behind catalaix goes far beyond experiments in the chemistry lab, and the aim is to test the real-world potential of the ideas and developments as quickly as possible. “RWTH Aachen University offers ideal conditions for the translation from basic research to developing a prototype,” Klankermayer says. “Thanks to the quality of process engineering and technical infrastructure here, we can carry out tests on a scale that would be unthinkable in our regular lab.” To ensure catalaix has the greatest possible impact, the project team also includes experts in the fields of sustainability and system evaluations who assess which newly created molecules are sustainable, how material flows might look, and where there is demand on the market.
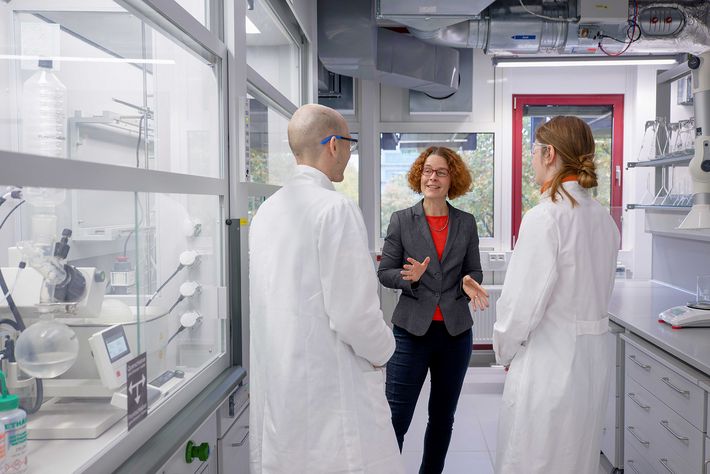
Platinum electrodes to break bonds
A tour of the Aachen labs reveals how the researchers are planning to realise their multilayered idea over the next ten years. To be sure, a considerable amount of preliminary work has already been done—for instance, the team have demonstrated that catalysis-driven bond splitting is feasible in several types of plastic. And in their research, the techniques they use to chemically break down plastic materials are as varied as the plastics themselves. For instance, in the labs where the research group of Regina Palkovits work, the experiments currently revolve around heterogeneous catalysis. This means the catalyst and the reacting substances in the reaction are in different phases—often the catalyst is a solid, while the reactants are liquid solutions.
The researchers are working on the idea of breaking down plastics through electrochemical catalysis, a somewhat neglected method whose great advantage is its sustainability: the reactions take place via green electric energy sources such as wind power rather than fossil energy. In the lab, the experiments are conducted using small glass containers with two metal platinum pins (electrodes) in the lid that go down far into the container. The container is then filled with a predefined type of plastic—the reaction medium. When voltage is applied to the electrodes, the reaction takes place on their surface.
Recently, the Palkovits group have demonstrated that the procedure can be used to split off polylactic acid (PLA), a chain-like compound consisting of the biodegradable molecule lactic acid that is used to manufacture packaging, cans and bottles. For their study, the researchers worked with a commercial bioplastic cup that they mechanically shredded and then placed in a solvent. As an end result, they achieved a maximum lactic acid yield of eighty-seven percent with the platinum electrodes. What’s more the conversion also worked on titanium, which is less expensive than platinum. Now, the team plan to find out whether this splitting method also functions with other kinds of plastics.
Reactions at 100 bar
In the labs of Jürgen Klankermayer’s group, the team are experimenting with what is known as homogeneous catalysts: catalysts and reactants that are in the same phase. With this approach, the aim is to break down plastics, in particular plastics from the polyoxymethylene (POM) class, which are used to manufacture zippers and backpack fasteners. POM is also important in the automotive industry, as it’s used to make door handles, rear-view mirrors and the buckles on seatbelts. In a first step, the researchers take these kinds of products as starting materials in their experiments and grind them in their in-house cryogenic mill, which has two balls that crush a material into a powder. The process takes place at low temperatures to prevent the mill from warming up, as heat can alter a material.
The plastic powder thus created is then poured into an autoclave—a gas-tight container used for chemical reactions. For the team’s initial experiments with a newly developed catalyst, these reactors are small, with a capacity of just a few millilitres. A magnetic stirrer mixes the catalyst and the plastic powder to trigger the reaction, generally at a pressure of 100 bar or more, which corresponds to over one hundred kilograms of pressure per square centimetre. In these experiments, Jürgen Klankermayer and his group were able to demonstrate that POM can be converted into so-called cyclic acetals when renewable diols—organic chemical compounds obtained from biomass—are added. For their part, acetals can act as solvents, polymer building blocks or pharmaceutical intermediates.
Another positive result in Klankermayer’s group was achieved in an experiment to break down polyethylene (PE), the world’s most commonly manufactured plastic. Used mainly for plastic bags and films, PE has a market share of some thirty percent. Together with a partner group, the catalaix researchers added biomass to polyethylene waste products to produce lactic acid, the building block of the bioplastic polylactic acid (PLA). In other words: the method developed by the Aachen team converts a conventional, non-degradable plastic into a biodegradable material that is suitable for a sustainable, recycling-based chemical industry of the future.


Looking inside molecules
Whether, how well and how efficiently these degradation processes function depends to a large extent on the catalyst that has been developed or selected for an experiment. These catalysts are often complex metal compounds, and even minimal adjustments to their chemical make-up can greatly accelerate or slow down a reaction. In addition, a catalyst’s form also contributes to its efficacy: in many cases, porous materials are suitable as solid catalysts due to the particularly large surface-to-volume ratio. The reason this is important is that catalyses generally occur on the surface of the catalyst.
This is also the reason it’s vital to understand exactly what the catalysts look like and how they work. A basement room at ITMC houses equipment that is essential for this purpose: seven vats of different sizes—so-called nuclear magnetic resonance spectrometers. Inside the instruments, a powerful and homogeneous magnetic field is applied to excite atomic nuclei, causing them to alter their state. “With this equipment, we can see the molecules, as it were,” Jürgen Klankermayer explains. And in the lab’s most modern spectrometers, reactions can even be carried out directly. The benefits are twofold: the researchers can see precisely what happens to their catalyst when it comes into contact with the reagent, and they can identify which bonds between individual chemical elements are formed or broken.
Microbes to build plastic molecules
Catalysis can also be carried out with non-chemically developed substances, referred to as biocatalysts. Indeed, many functions in the human body are impossible without biocatalysts—the microbes that inhabit our body—and people have been making commercial use of these biocatalysts for centuries to brew beer or ferment wine. Now, Lars Blank, Chair of Applied Microbiology at RWTH Aachen University and member of the catalaix core team, is applying the same principle and using enzymes to degrade plastic. In addition, his research group use genetic engineering to insert enzymes into microorganisms, which can break down specific plastic compounds or assemble them into larger molecules.
“Our approach is best suited for application on mixed plastics,” Blank says. Using several different enzymes that other research partners have contributed to the catalaix project, mixed fragments or microplastics from the environment can be broken down into various kinds of monomers (molecules that can be bonded to other identical molecules to form polymers). Blank’s research group is already testing these systems in so-called fermentation apparatuses: within just a few hours, pieces of plastic are converted into aqueous solutions. This interplay between chemical catalyst and biocatalysis represents additional possibilities for researchers in the catalaix project to chemically degrade plastic into its monomers. In a further step, Blank envisions that microbes will absorb these monomers and assemble them into biomolecules. “This means we’re creating new building blocks and polymers at a place that gets very complicated for chemists, because they would have to run various reactions one after the other or under different conditions—or because the substrates are impure or their composition is unstable,” Blank explains.
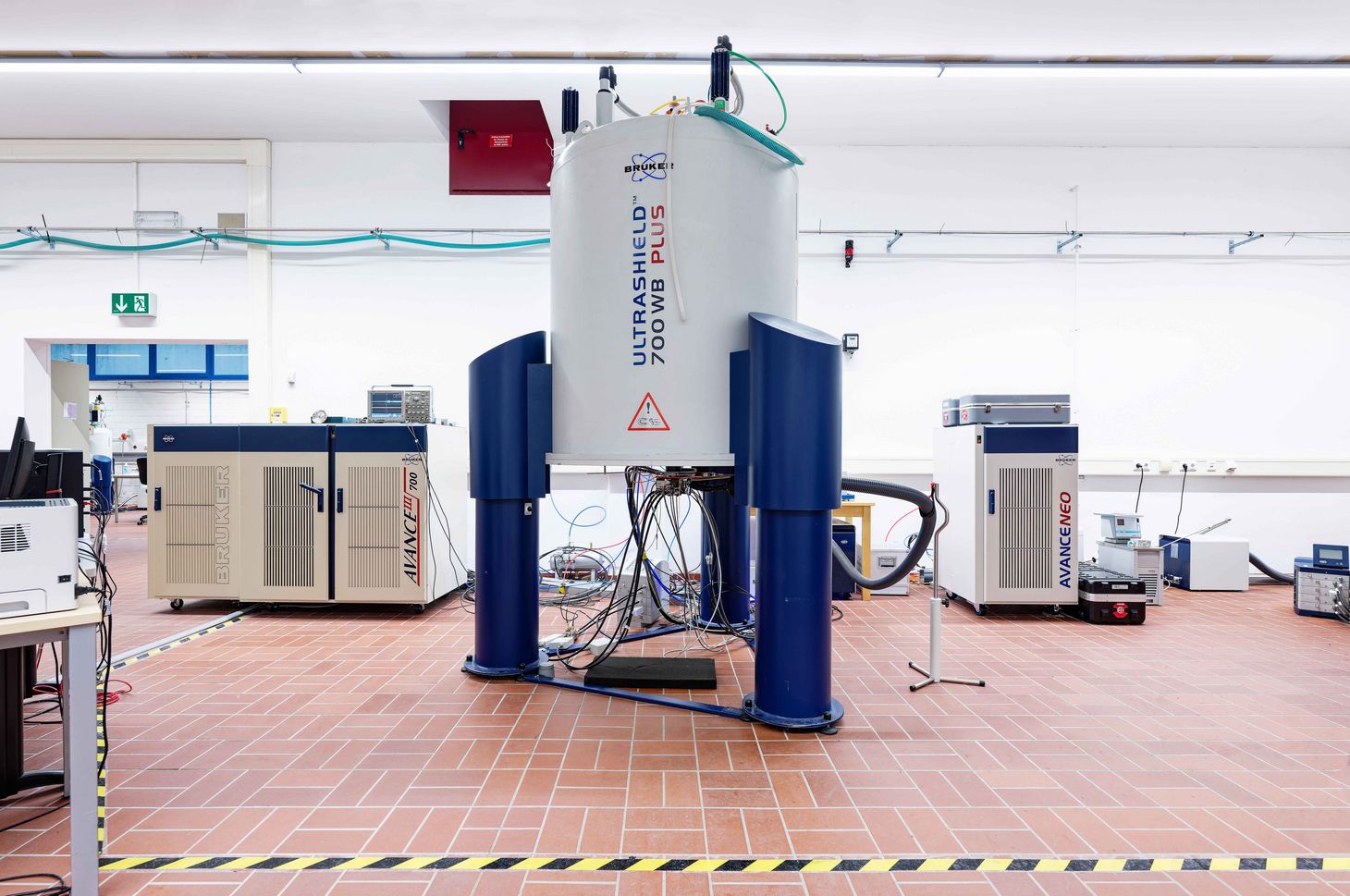
Everything can change in the reactor
To attain the project aims, however, lab results demonstrating that a plastic compound can be broken down using catalysis or a specific enzyme are just a first step. It’s at least equally as challenging to translate these findings into a method that businesses can use to recycle the kind of waste products consumers throw away every day. This is why the catalaix team want to transfer their findings from the lab to real-world applications as quickly as possible.
Polyethylene is an example of one such process. At the “Technikum” in Aachen, directly adjacent to the ITMC building, the researchers have ideal facilities for conducting larger-scale experiments. Diverse high-
pressure chambers are located along a narrow corridor, and in one of the chambers, a PhD student has installed a complex reactor unit that is roughly two metres wide; inside the reactor, polyethylene admixed with ethylene is broken down via a catalytic reaction into propylene, the basic building block. This work is the continuation of an experiment on plastic degradation that was recently concluded in the ITMC lab. However, rather than holding just a few millilitres, the autoclaves at the Technikum have a capacity of one litre.
Volume, however, is not the only difference between experiments conducted in the ITMC lab and those at the Technikum. A particular problem is that the parameters of an experiment change with the size of the autoclave. For instance, the agitator to mix the materials is different, the flow conditions shift, mixing can become more difficult. The goal is to find out whether new problems arise due to the larger scale. But that’s not all: with an eye to a later industrial application, the researchers are already taking initial measurements to learn whether the method has the potential to be economically viable. How much substrate can be converted? What temperatures and pressures deliver the best results? What are the precise reaction conditions?
Maintaining constant processes
Answers to these questions are needed for the next stage: building a pilot plant that will be operated by process engineers—and that will mark the transition to production on an industrial scale. This step, too, was part of the original catalaix concept, as process engineering plays a pivotal role in the project. Unsurprisingly, it’s also only a short walk from ITMC to the Aachen process engineering building, where testing units that can convert volumes of fifty to one hundred litres are being built. In the experiments conducted here, the main focus is to go beyond processes conducted under ideal conditions. “We’re also looking into aspects like control systems—in other words, how we can run these processes in such a way that overall quality is guaranteed even when certain dimensions or the make-up of the input product change,” says Alexander Mitsos, who is Chair of Process Systems Engineering at RWTH Aachen University—and also a member of the catalaix core team.
Mitsos’s research group are focused on developing methods and algorithms to optimise chemical and electrochemical processes, enabling them to make predictions that are of particular benefit to other researchers in the project. For instance, Mitsos and his team have already used machine learning to predict the fuel properties of molecules on the basis of their atomic bonds.
The potential challenges arising in the catalaix project on the journey to developing a marketable chemical-
catalyst system are also seen in the biorefinery operated at Aachener Verfahrenstechnik—the building for systems engineering containing several demo units in which processes based on renewable raw materials are run and tested on an industrial scale. In one of the demo units, lignin—a key component of wood—is currently being degraded. In these experiments, details that might seem negligible in an ordinary chemistry lab can balloon into major stumbling blocks. For example, sometimes steel containers are damaged by reactions when a corrosive reagent is added. And sometimes solvents used to work on solids turn out to be too expensive in the amount needed for large-scale operations.

Life cycles and value chains
The data provided by the process engineering studies represent yet a further step in the team’s journey towards a successful product launch: acquiring an in-depth understanding of operational aspects. This includes investigating how a new plastic or molecule is fabricated, how much energy and resources are needed for production, and what the corresponding value chains look like. These are some of the questions that Grit Walther and her group must answer in order to do their job. Walther is Chair of Operations Management at RWTH Aachen University, and she, too, is a member of the catalaix core team. Her specialisation lies in modelling and assessing production and value chains.
The analyses she conducts are often complex and highly intricate—for example, in cases when the task is estimating a product’s impact on and in the environment on the basis of its life cycle. For this, she draws on criteria such as carbon emissions, acidification, eutrophy cation, land use and water consumption. “Sometimes,” Grit Walther says, “we also work with social criteria.” If, for example, a business collects plastic waste in Asia, she and her team study how jobs and the general levels of acceptance in the region are impacted if waste purification occurs in Europe rather than locally.
That the ten-year duration of catalaix is adapted to the challenges is important to Walther—and also that the chemists in the groups led by Jürgen Klankermayer and Regina Palkovits have prepared several products that are already being tested on a larger scale. “The more scaled their findings, the better our predictions,” is how she summarises the connection. “If we only have lab data, the unknowns in our calculations are very large.” However, the path from lab to modelling and assessing is a two-way street, an iterative undertaking, as Grit Walther explains: “Based on the data we get from the lab and from process engineering experiments, we create models—and then feed them back to the researchers, who in turn adjust their experiments to reduce environmental impact or improve marketability.”
This kind of interactive collaboration is more or less the essence of catalaix: innovative research calls for teamwork, and in order to make key advances in science and society, it’s critical that experts from various disciplines work together and that they respect, trust and support one another. Over the next ten years, the WSS Research Centre in Aachen will serve as a prime example of how this kind of modern collaboration delivers results.
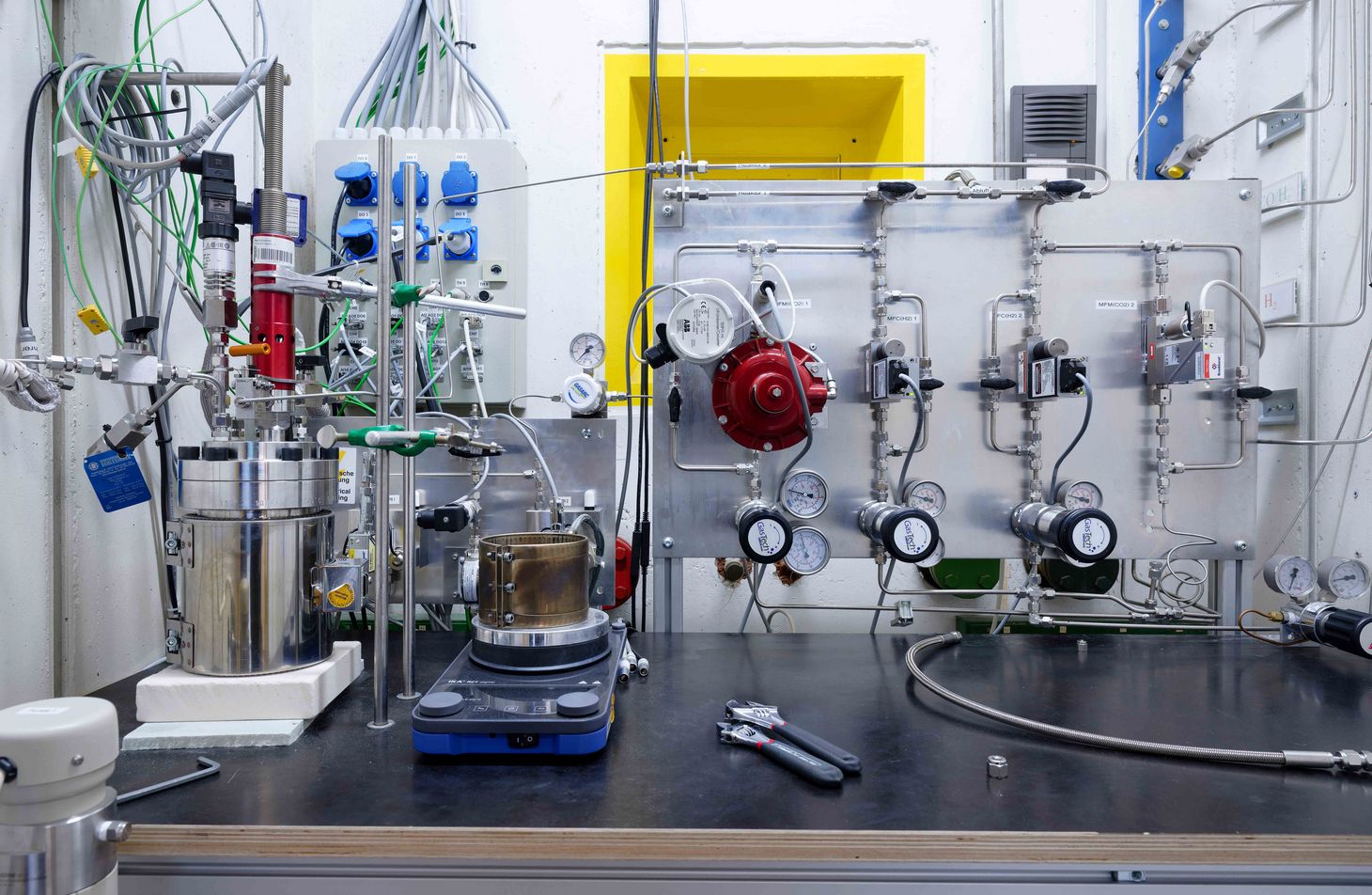