News
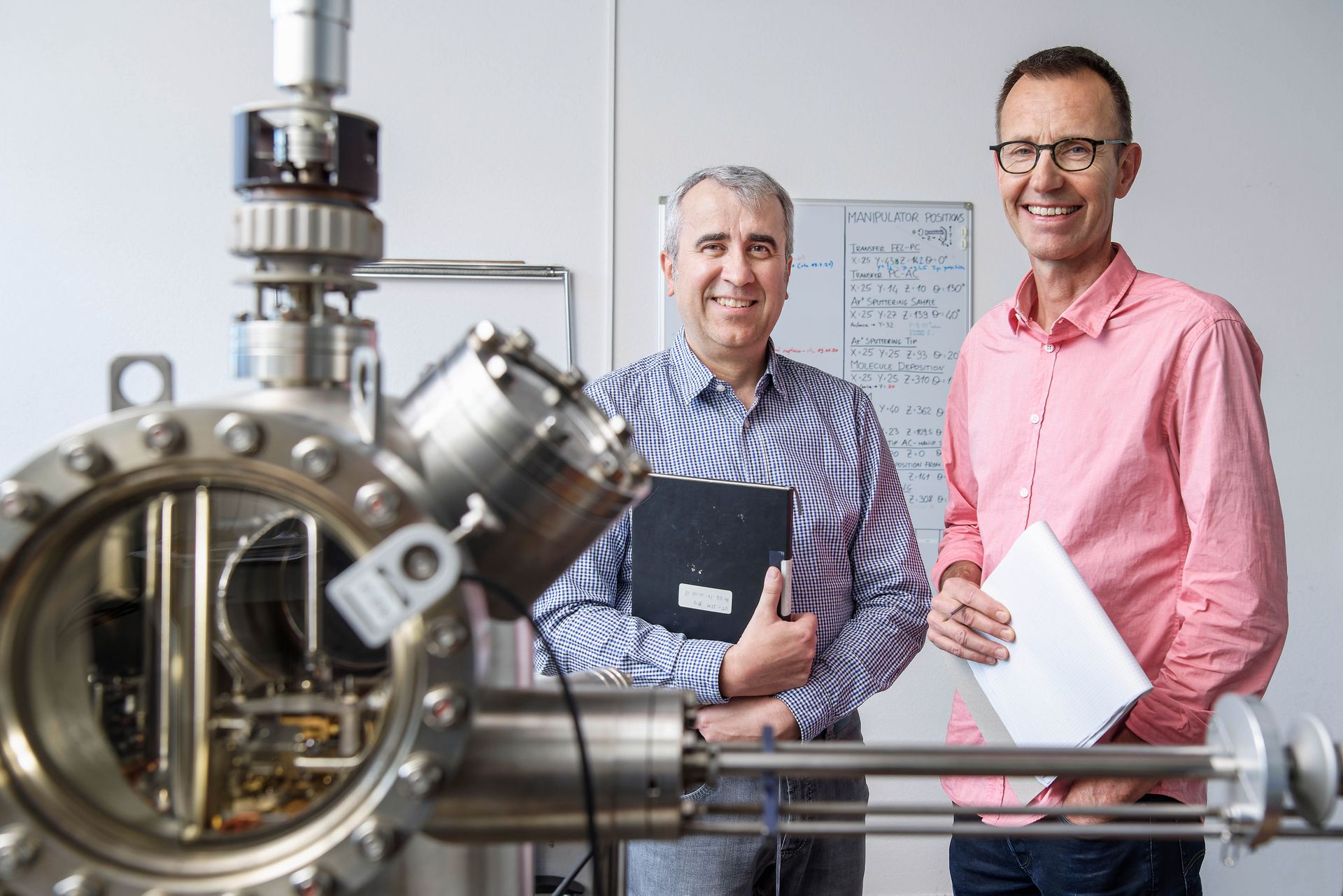
Wanted: gigantic computing power
The race to develop new nanomaterials for quantum technology is in full swing. Participants include the CarboQuant team as well as numerous other researchers and companies throughout the world. How does the Empa CarboQuant project in Switzerland differ from the rest, and what are its objectives? A discussion with Roman Fasel, project leader and director of the nanotech@surfaces Laboratory, and deputy project leader Oliver Gröning.
Physicists and engineers across the globe are on the hunt for quantum effects. Why the hype?
Roman Fasel: Quantum technology is simply the last real frontier remaining in electronics, and the enormous advances made in nanotechnology in the recent past mean that entering the realm of quantum technology is within reach. It’s probably this pioneering spirit that has driven much of the research. And thanks to this progress, we’re now able to construct the complex structures of our nanoribbons with such precision that we can exploit and manipulate quantum effects—and that on a scale where just a single atom makes a huge difference. It’s impossible for electronic components to go into greater detail.
When quantum technology is talked or written about, quantum computers and the anticipated explosion in computing power are generally the issue. But why do we need more computing power?
Fasel: That’s a fair question. And it’s true that, over the past fifty years, computers have become increasingly powerful, even with today’s semiconductor technology. Just imagine: today, every single smartphone has more computing power than was used for the moon landing. But there are still many problems our computers can’t yet solve.
For example?
Fasel: One example would be weather forecasts that are accurate for more than just a few days. Another would be more precise global climate prognoses. For more exact predictions, the models that describe these phenomena need to be calculated in much greater detail and on a much smaller scale. But when calculations are made on a smaller scale, the computing capacity required increases exponentially. Today’s computers quickly reach their limits. Other science disciplines, or the financial sector, would also benefit from more exact models.
Oliver Gröning: This is precisely where quantum computers come in: with each additional qubit, computing power increases exponentially. In conventional computers, by contrast, processor speed increases on a linear axis. And there we’ll always lag behind when it comes to challenges involving exponential scales. These problems can only be addressed by a computer capable of exponential scaling: a quantum computer.

In your search for the right materials for quantum electronics, you’ve decided to focus on structures made of graphene—a material consisting solely of carbon. Isn’t that a major limitation?
Gröning: No, because carbon is an extraordinary element. About eighteen percent of our body weight is made up of carbon, and every organic molecule is carbon based. This is because the carbon atom can form a wide range of different chemical bonds. Sometimes it has four neighbours, sometimes three, sometimes two. In molecular structures, carbon can combine to form one-dimensional chains, two-dimensional planes or, as in the case of the diamond, ordered three-dimensional structures. No other material has this structural flexibility. With carbon, we can design multiple new geometries.
How do you do this?
Gröning: We reverse previous approaches. Until now, researchers have generally begun with the material. In experiments or computer simulations, they then study specific materials to learn about their interesting properties. We propose doing the opposite. First, we define the properties we want to have and then look into what structures could have these properties and how we could design corresponding structures.
Fasel: To realise our idea, we want to develop a technology platform where nanoribbons, as the basic material, are available, as are the expertise and the infrastructure necessary for targeted further development. We’ve built up the basis at Empa over the course of the past twelve years. We can now create precisely designed graphene nanoribbons, and we’ve developed an understanding for how we have to change their structures to control quantum effects. We now want to expand this foundational knowledge into a platform.


What are the first steps to creating a platform?
Gröning: To begin with, we want to generate a larger library of different nanoribbon structures and establish a well-functioning infrastructure and methodology to characterise and further develop the nanoribbons. This calls for a lot of detailed groundwork that may not immediately deliver spectacular results, but which is indispensable for making progress.
Fasel: This is another reason why our project needs long-term funding that goes beyond the usual two- or three-year grants awarded to individual projects. It’s like the discovery of the continents hundreds of years ago. If you only sail from port to port, you’ll never cross the ocean. For that, you’ll need a large ship with sufficient provisions—enough that you don’t face starvation at the first calm. Today, we don’t know for sure where the journey will lead us. But Magellan didn’t know that either when he set sail in 1519 to find the western route to the Spice Islands.
The competition is fierce, with companies like IBM and Microsoft investing billions in the development of their quantum technologies and quantum computers. Can you hope to keep up?
Fasel: No, but that’s not our goal. In addition to our carbon nanoribbons, there are indeed many other approaches being pursued, some of which are already quite far advanced. For example, extremely sensitive measurement sensors based on quantum effects have already been developed, and we have the silicon-based or superconducting qubits that were used to construct the first quantum computers. But all these approaches draw on phenomena that occur only at extremely low temperatures close to absolute zero. This is also why they need gigantic and horrendously expensive cooling systems that rely on liquid helium.
By contrast, our carbon nanoribbons are stable at normal ambient temperatures. As such, it’s not the first generation of quantum technologies that interests us. We’re specifically working on the second generation: a quantum technology that doesn’t require huge, costly cooling systems, that consumes less electricity and that’s based on carbon—an element that is non-toxic, inexpensive and widely available. In short, we’re looking to realise a quantum technology that at some future time will better serve our everyday needs.
Interview: Santina Russo
Photos: Felix Wey