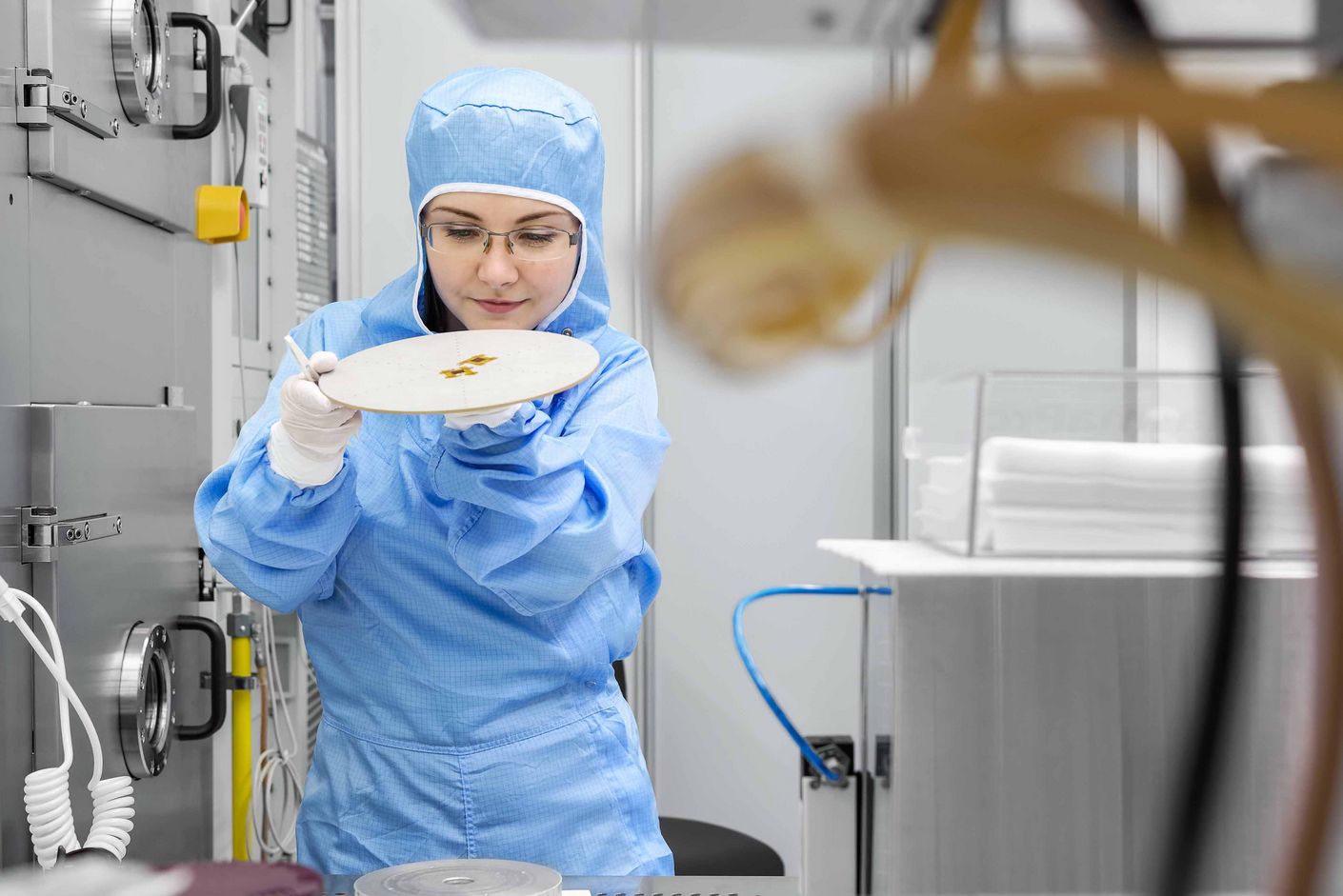
The power of a single atom
Computers, USB sticks, smartphones: every electronic device contains millions of energy-hungry components. The team at the Center for Single Atom Electronics and Photonics are developing highly energy-efficient components using a principle based on the switch function of a single atom. Now, the team has once again topped their own world record in energy efficiency.
“Imagine we had a microchip that was a thousand times more efficient than today’s computer chips,” says Professor Jürg Leuthold, director of the Center for Single Atom Electronics and Photonics at ETH Zurich. Developing precisely this kind of chip is the goal he is pursuing with his project partners, Professor Mathieu Luisier of ETH Zurich and Professor Thomas Schimmel of the Karlsruhe Institute of Technology. A novel principle is at the root of their approach: instead of utilising the flow of electrons as is common in standard electronics, their components are based on the switch function of a single atom. The idea is to place metallic contacts (electrodes) close together—so close that the space between them is the size of a single atom. When an atom is shunted back and forth across this miniscule gap, an electric current either flows or is disconnected. A certain degree of activation energy is required for each individual switch. When applying the single-atom principle, however, this amount is negligible: the latest version of the single-atom transistor constructed by Thomas Schimmel and his team consumes a mere three millivolts of activation energy—a world record—and the previous model was already hyper-efficient, requiring only six millivolts. “We attained these significantly better values last year by optimising the construction of the electrode microstructures,” says Schimmel. As a comparison: standard transistors used in devices like smartphones consume nearly a volt of electricity—300 times more than the single-atom transistor. “Once the system is ready for market, we expect energy efficiency to be as much as 1 000 times better,” says project leader Jürg Leuthold.
From gel to glass
To date, the clever principle functions only under laboratory conditions in a system that uses a gel electrolyte as a charge carrier. Because gel electrolytes are difficult to realise in CMOS devices, Jürg Leuthold’s research group is exploring ways to apply the single-atom switch function in a system made of solid materials. The researchers recently took a major step towards this goal by constructing a single-atom component with platinum and silver electrodes and using glass as a charge carrier in place of the gel electrolyte. The researchers then connected the single-atom component to an inverter, a key building block in common electronic devices. This led to a surprising discovery: with this combination, what is known as “dark current” is 100 times lower. Dark current is the amount of electricity that electronic components consume even when they are switched off and that collects in electronic devices in the form of standby power, also called “vampire power”. In addition, Leuthold’s combined set-up reduces power consumption by a respectable factor of 10 when the component is switched on.
Handy computer simulations
At the same time, the team are seeking the best materials for constructing the electrodes. In the gel system, silver proved most advantageous—due to its electronic properties and because the malleable material proves ideal in forming the minute electrode structures. To further optimise their system, the researchers have begun working with computer simulations. “It would be impossible to test all the different materials in lab experiments,” says Leuthold. The reason is that an extremely wide range of materials could theoretically be used as a charge carrier for the electrodes (including gel, glass, titanium oxide or aluminium oxide) and because the potential production processes are equally numerous. This is where the group of Mathieu Luisier enter in: they developed a computer program modelled on the physical laws and properties of atoms to simulate how a material would perform in the single-atom system. Last year, the researchers further developed and validated their algorithm on the basis of the current single-atom transistor with the tin electrodes. “In future, we can use the simulations to examine different materials and to keep learning more,” says Leuthold.
Text: Santina Russo
Photo: Felix Wey