News
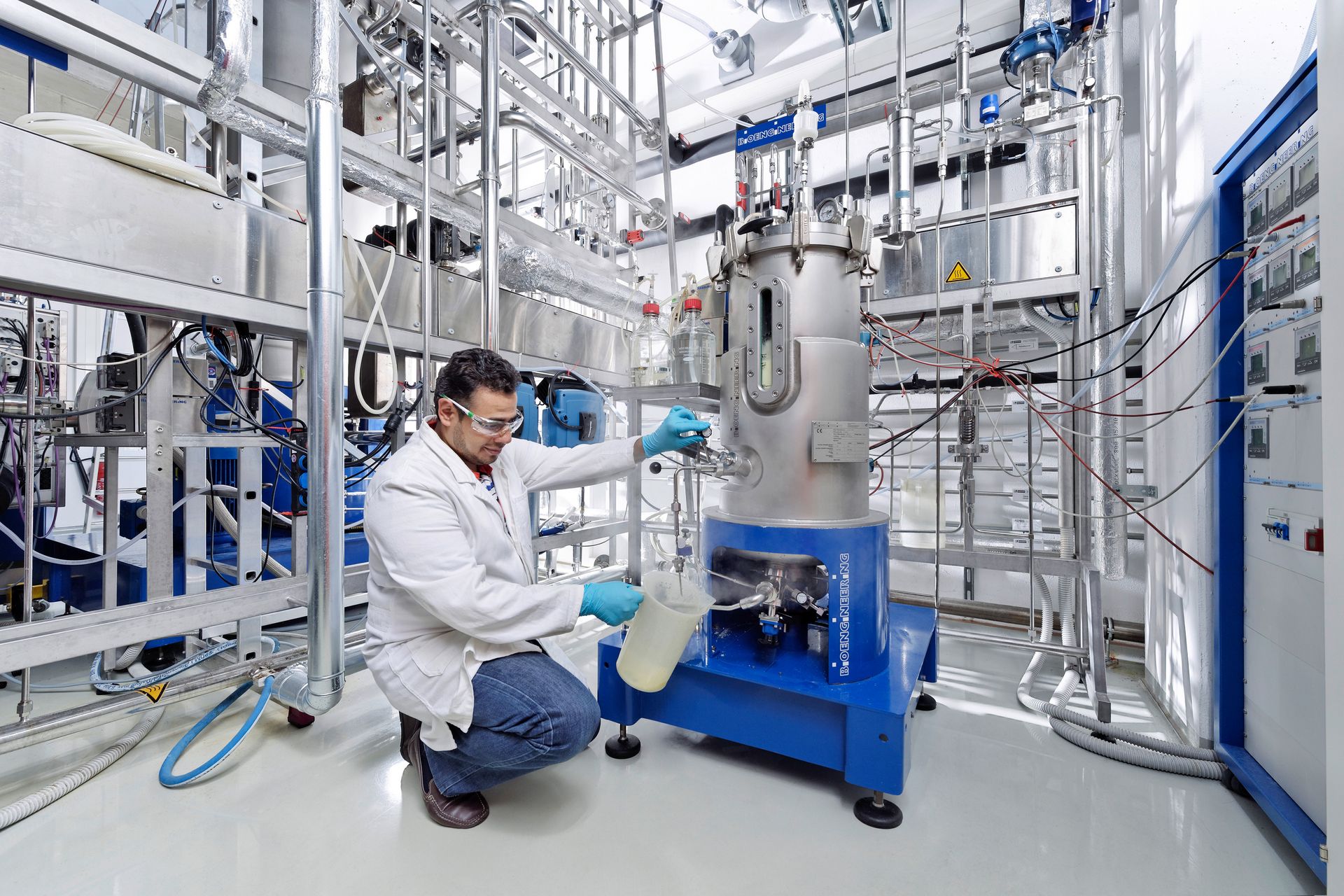
Yeast and algae
One of the breakthrough discoveries for Thomas Brück and his team are yeasts that, during their growth stage, metabolise carbohydrates (sugar) into energy-rich oils rather than alcohol (ethanol), as is normally the case. This feat is accomplished by the yeast Trichosporon oleaginosus, which was isolated from a compost heap in Ireland. It is a highly unconventional yeast that can convert diverse carbohydrates into biomass and special oils. With up to 40% fatty acids, the oils produced resemble olive oil.
Trichosporon oleaginosusisn’t too choosy when it comes to food: it feasts on the waste of corn, crabs, wheat bran, wood shavings or straw, pulling out every convertible carbohydrate. “The yeast can be added to all manner of existing waste products, which ensures that production is sustainable,” says project leader Daniel Garbe. In a series of chemical metabolic steps, the yeast converts the carbohydrates into fat that it can fall back on in lean times—much like our own “padding”.
Stress triggers fat production
Normally, a single yeast cell consists of 20% fat, but in times of stress, it increases its fat reserves up to 80%. The researchers take advantage of this reaction and put the yeast on a “diet”: during its growth phase, the yeast is deprived of nitrogen and phosphate. The yeast reacts by using all its remaining energy to create emergency fat reserves. “When we view yeast under a microscope, we basically see only fats and a few cell structures,” says Thomas Brück. When the yeast reaches this point, the researchers end the “diet”. This ensures they have the highest yield because the “hungry” yeast hasn’t yet begun to resort to the fat stores for its own survival.
Profiling fatty acids
By applying the art of chemistry, fats can be transformed into just about anything. One of the research teams in the Synthetic Biotechnology group is now experimenting with a variety of fatty acid “profiles”. The team changes various parameters and observes how the yeast reacts. For example, when the researchers feed the yeast different kinds of sugar, the fatty acids produced have different profiles. And a similar effect on the profile of the fatty acids can be attained by varying the temperature or by removing sulphureous substances from the culture medium. These manipulations prompt the versatile yeast to release a different profile of the fatty acids.
How is this possible? Chemically speaking, the different fats and oils are related: they’re structured around a glycerol molecule joined to three different fatty acid units having a different number of carbon atoms. This gives rise to the term “triglyceride” for biologically produced fats and oils. Oils used as food also have many double bonds, in which case they are called polyunsaturated fats, whose antioxidant properties render them very healthy. Depending on the profile, various kinds of oil can be made from the fatty acids: oil for food (palm oil alternatives, fish oil derivatives, Omega-3 fatty acids for baby food) or the oily base for biofuels, plastics and cosmetics.
Alternatives to palm oil
To date, many plant oils are harvested from plants that are grown in environmentally detrimental monoculture and that damage or destroy the ecosystem. To produce palm oil, valuable rainforests have been cut down, depriving endangered animals such as the Orang-utan of their habitat. Palm oil, in the meantime, is an ingredient in numerous food and cosmetic products—and despite its non-ecological production, demand remains high.
“The composition of fatty acids in palm oil unfortunately can’t be produced naturally in other organisms.” This is how Norbert Mehlmer, genetic engineer at Synthetic Biotechnology, frames the problem. To find an alternative to palm oil, the researchers are resorting to new methodologies to insert a foreign gene into yeasts. As a first step, the researchers use the soil bacterium Agrobacterium tumefaciensas a door opener for the foreign gene. In a second step, the yeast culture is then treated with an antibiotic, causing the Agrobacterium tumefaciensto die. What remains is yeast with the desired genetic make-up able to produce tailor-made, high-quality fats and oils—safely, year-round and reliably.
The industrial partners of Thomas Brück’s team at Synthetic Biotechnology see great potential in two other fatty acid profiles: fish oil alternatives and linoleic acid, an Omega-3 fatty acid for baby food. “In the next five to seven years, these two oils could be ready for commercial production,” Brück says.
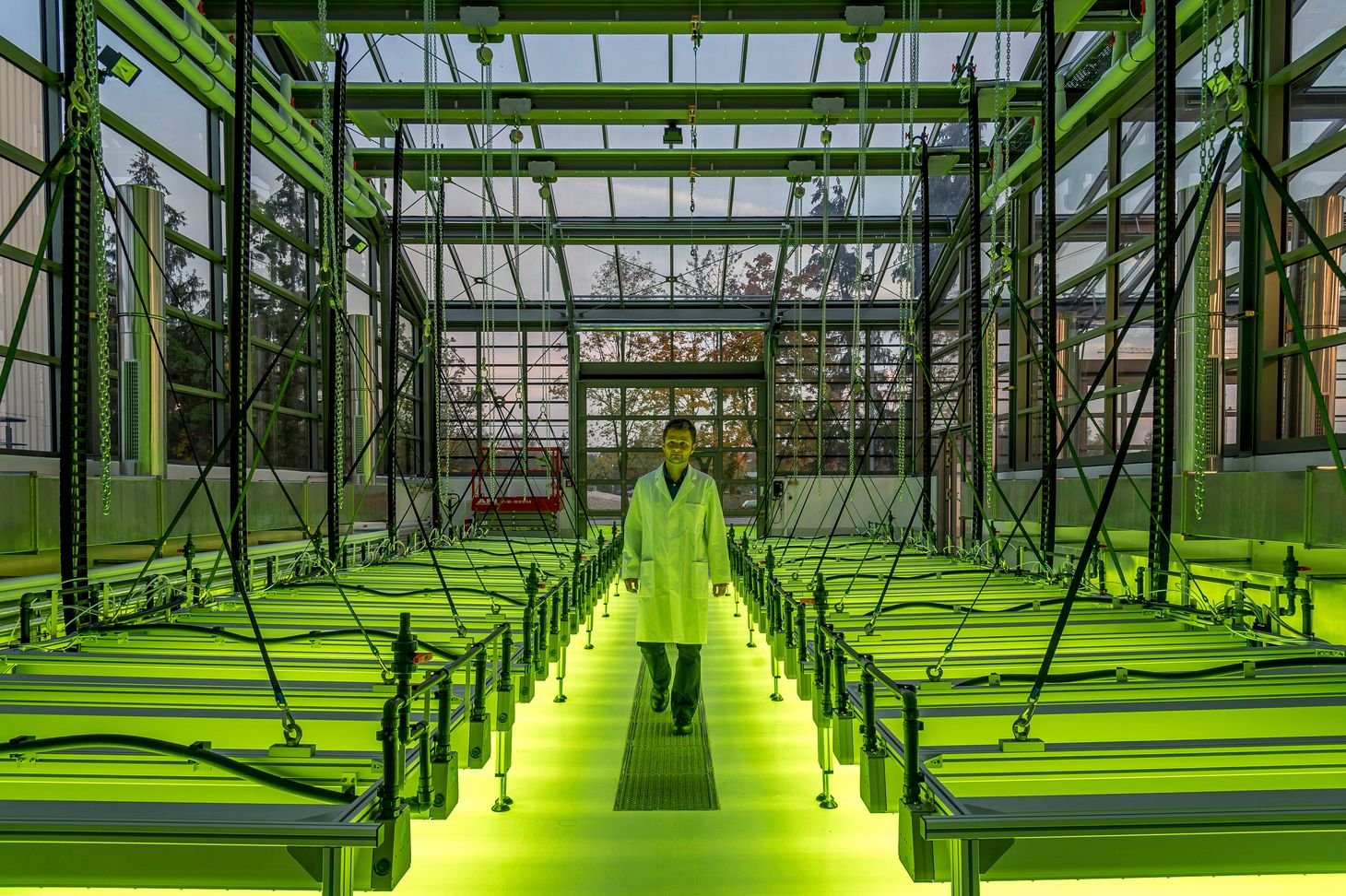
Plant oil as a raw material
The team at Synthetic Biotechnology believes it can help to solve the issues surrounding the potential shortage of raw materials. The raw material “plant oil” is exemplary of the almost intractable problem: already today, 30% of all plant oil is used to generate energy and manufacture chemicals. As a result, it’s not available for use in the food industry. Premium vegetable oils are converted into biodiesel, soaps (surfactants) or other oily chemicals that can be used in products such as car lubricants and body lotions. Thomas Brück is convinced: “Plant oils should be used for foodstuffs, not to produce energy or chemicals.”
Plastic made of crab shells
Thomas Brück and his team are also working on a project to manufacture sustainable plastics (polymers). The raw material here is once again entirely natural: empty crab shells from Ireland. The Irish distributor was more than happy to deliver the waste product to the research team: because crab shells attract bacteria that produce poisons, they must not be disposed of with normal waste. Proper disposal costs 7,500 Euro per metric ton.
The TUM research team worked with partners from Germany, Czechia, Norway, Austria, Tunisia and Indonesia to develop a way to manufacture high-quality plastic from old crab shells. To do so, they first dissolved out the polysaccharide chitin from the crab shells. Then, they optimised the fermentation process and genetically altered the lipids until they could be used to manufacture a particularly high-quality polyamide. Evonik Industries was the industrial partner in this step. Medical devices, including dental and bone implants, can be produced using the polyamide. “This project was a great success,” says Thomas Brück.
Fighting cancer with bacteria
In another group, project leaders Monika Fuchs and Norbert Mehlmer focus on relaying new biocatalysts in the metabolism of gut bacteria Escherichia colito ensure the sustainable production of medications such as the tumour drug Taxol. Until now, the clinically valuable agent to combat tumours has been produced from plant extracts in a series of chemical synthesis steps. And for every kilogramme of the agent won, another 120 kilogrammes of waste are generated. The methodologies developed at Synthetic Biotechnology allow the team to construct a genetically tailor-made cell factory capable of manufacturing the desired product without generating toxic waste. The genetic changes in individual biocatalysts in the cell factory also open the door to creating even more new agents that function in anti-inflammatory drugs or that can be used in biodegradable insecticides.
Flying high with algae
The doctoral students under project leaders Farah Qoura and Daniel Garbe have been working intensely for three years on generating fuel from algae—as an alternative to kerosene. Flying with algae—an enticing thought. And there are many good reasons to be optimistic: algae grow 12 times faster than terrestrial plants and require neither freshwater nor cultivated land to thrive. Due to their fast growth, algae are efficient consumers of the greenhouse gas carbon dioxide, which they transform into biomass and biogenic oils.
The first positive results have been secured: the researchers located various salt-water algae in Australia, Mexico and the Adria that have the potential to produce oils. In the lab, the team exposed the algae to UV rays to isolate new genetic stems that also thrive in very salty water and that are distinguished by a very high oil production. The oils produced by these algae provide the starting point for producing alternative biofuels. Two types of algae are currently the most productive: Nannochloropsis salinafrom the Caribbean and Dunaliella sp.from Australia. Both kinds thrive in extremely salty waters, where the alkaline environment protects them from terrestrial contaminants such as fungi and bacteria—a further advantage over freshwater algae.
Text: Brigitt Blöchlinger
Photos: Felix Wey