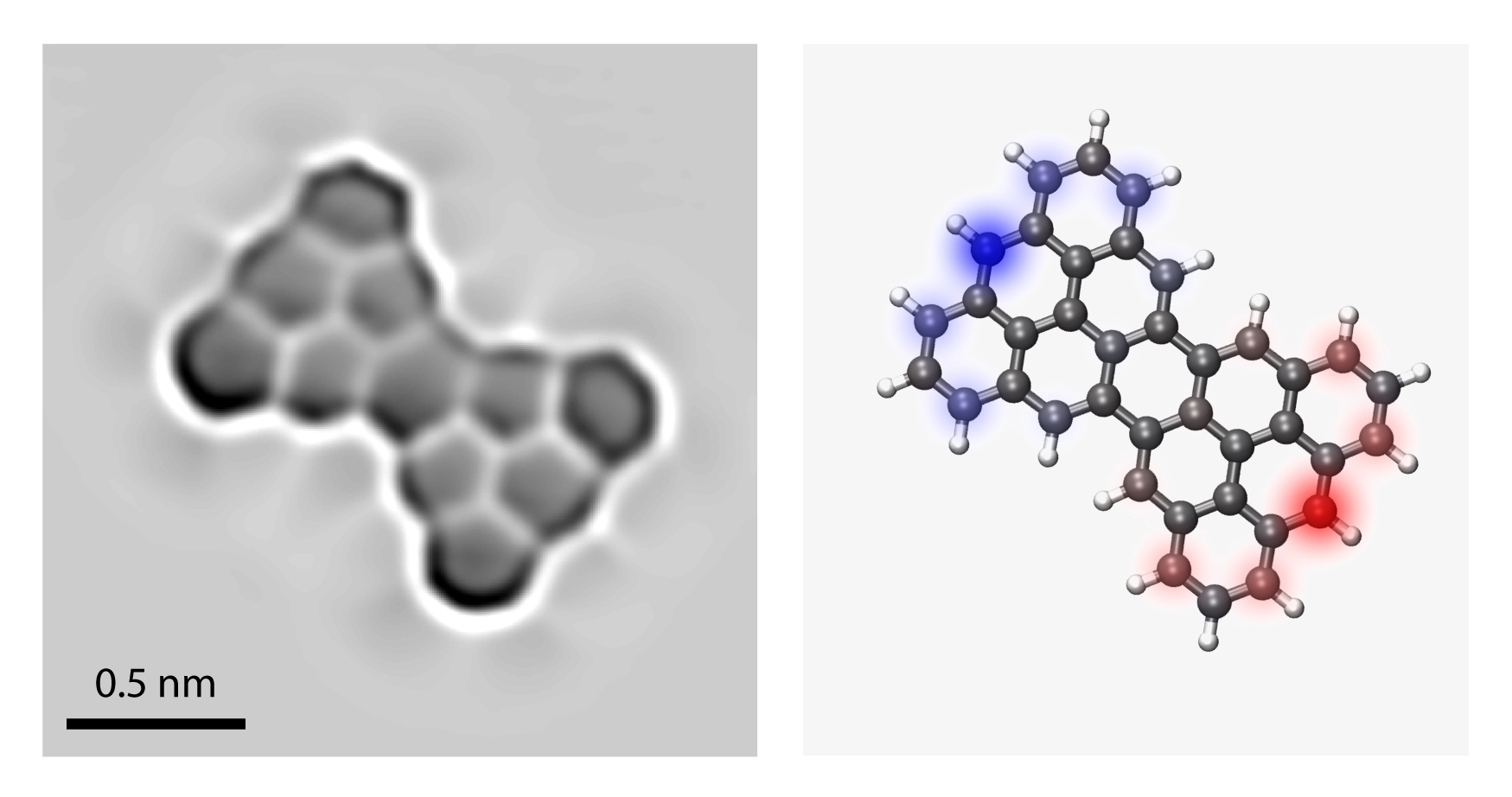
Spin chains made of carbon
In the CarboQuant project at Empa, researchers are using carbon nanostructures to study quantum physical phenomena. They’ve now achieved a breakthrough by precisely constructing and testing one of the most important theoretical quantum models.
The field of quantum physics promises to bring about revolutionary new technologies—for example quantum computers capable of performing calculations that are too complex for even the most advanced supercomputers. However, the behaviours of, and interactions between, elementary particles and atoms—the subject of quantum physics—are complex, fragile and often strangely contradictory when considered using ordinary human reasoning.
One example of these unusual traits are superposition states. The smallest unit of information in conventional computers, the bit, can have either one of two states: on or off, represented by a zero or a one. By contrast, the qubit, the unit with which quantum computers calculate, can be a zero or a one—and also any combination thereof, making a theoretically infinite number of states possible. This capacity is what enables quantum computers to carry out not just one calculation at a time but several calculations simultaneously.
Many systems and methods in physics could potentially be used for building qubits. In the WSS-funded CarboQuant project led by Roman Fasel and Oliver Gröning at the nanotech@surfaces Laboratory at Empa in Dübendorf, Switzerland, researchers are working with one of these possibilities: they develop carbon nanomaterials with atomic precision and then use their “nanographenes” to study spin effects.
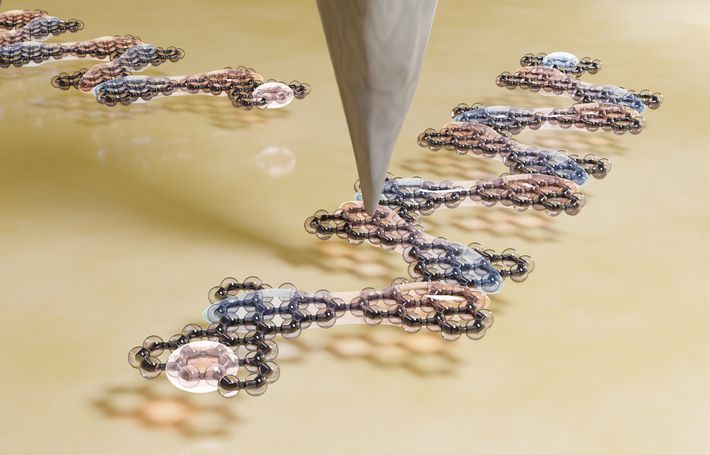
Building set for quantum spins
The spin is one of the fundamental quantum mechanical properties of charged particles such as electrons and protons. Put in simple terms, a spin is a magnetic moment arising from an angular momentum that indicates two directions: up (state one) and down (state zero). If two or more spins are linked, they exercise a mutual influence on each other: for instance, if the direction of one spin is altered, that of the other spins will also change.
The interactions are incredibly complex. To be sure, mathematical models have been devised to describe them, but these equations are rendered nearly unsolvable already in even relatively simple spin chains. Moreover, quantum mechanical phenomena take place at the sub-atomic level, making it yet more difficult to realise—that is, build—these spin chains.
Over the past fifteen years, the CarboQuant team have perfected an elaborate synthesis with their carbon-nanomaterials approach. “We’ve compiled a type of modular construction kit for quantum spins,” Oliver Gröning says. “This means we’re now able to predict how a molecule should look on the basis of the desired spin properties—and then to fabricate it.” And Roman Fasel says there’s more: “With our expanded repertoire, we also have the ability to turn the spins on and off with precision.”
From theory to reality
Recently, the researchers published a study in top-tier journal Nature Nanotechnology (*) detailing how they produced and precisely measured the properties of a theoretical quantum mechanical model of a spin chain—the one-dimensional alternating-exchange Heisenberg model. The Heisenberg model is a linear chain of spins in which each spin exhibits a strong interaction with one of its two neighbouring spins and a weak interaction with the other.
To create their Heisenberg model, the CarboQuant researchers and their colleagues from Braga, Portugal, and Dresden, Germany, used “Clar’s goblet”, a molecule shaped somewhat like an hourglass and consisting of eleven carbon rings. The molecule was named in honour of German chemist Erich Clar, who more than fifty years ago predicted that each end of this two-dimensional carbon compound contains an unpaired electron with its own spin. Clar, however, never managed to transform his theoretical model into a physical molecule. Nor did anyone else—until five years ago, when the team led by Roman Fasel and Oliver Gröning succeeded in producing Clar’s goblet and demonstrating its spin properties.
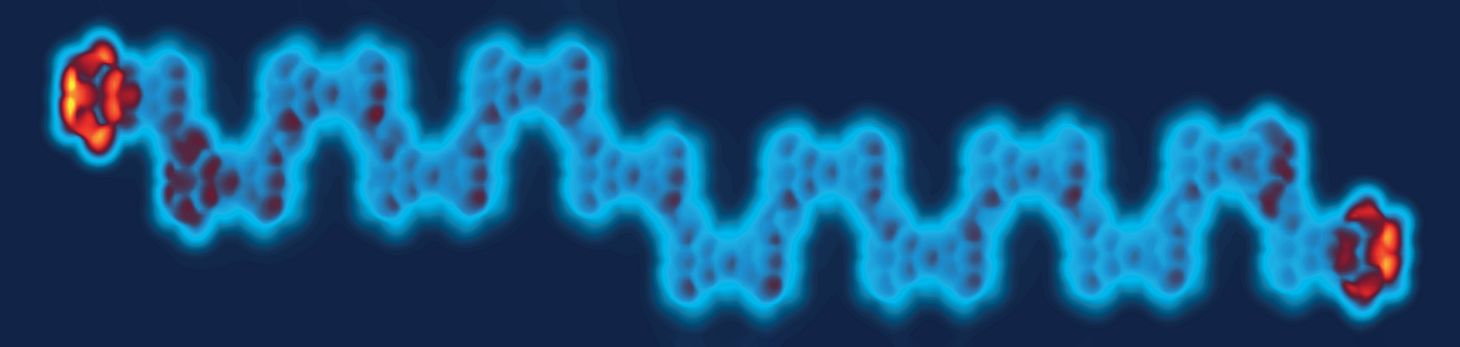
Coupled and measured
For their latest publication, the researchers went a step further by linking the goblets to a gold surface to form chains. This brings about the precise spin interactions predicted in the Heisenberg model: the two spins within a single molecule exhibit a weak interaction, whereas the spins between two neighbouring molecules are more strongly coupled.
Using a scanning tunnelling microscope, the CarboQuant team produced precisely defined spin chains of varying lengths. To study them, the researchers pinpointed individual spins, switching them on and off—or “flipping” them from one state to another. “The way we measure the magnetic properties of our chains at different spin sites is like studying flow patterns at different positions on an airplane model in a wind tunnel,” Oliver Gröning explains. The approach enabled the team to confirm various theoretical predictions, including the following: free electron spin states form at the end of longer, but not shorter, chains.
Roman Fasel says the study findings open up new possibilities in quantum research. “We’ve now demonstrated that theoretical models in quantum physics can be realised using nanographenes, and that the models’ predictions can be tested experimentally. Nanographenes with other spin configurations can be linked to form other kinds of chains or more complex systems.”
Two state-of-the-art microscopes
The researchers are already planning their next steps. “Put simply, our current instruments enable us to switch an individual spin at a specific site completely from ‘down’ to ‘up’,” Oliver Gröning explains. “Now, however, we don’t want to reverse the spins completely. We want to switch them ‘halfway’.” This is critical, because only half-switched spins will generate the spooky superposition quantum state in which they simultaneously point up and down. This capacity would open the door to performing quantum operations.
The CarboQuant researchers want to achieve their lofty aim with the two new, cutting-edge scanning tunnelling microscopes now being set up in the nanotech@surfaces Laboratory. Financed in part by the Werner Siemens Foundation, the microscopes function at low temperatures with extremely powerful magnetic fields and radio frequency signals—the precise prerequisites for manipulating and measuring the magnetic properties of carbon nanomaterials with ever greater precision.
> (*) Nature article
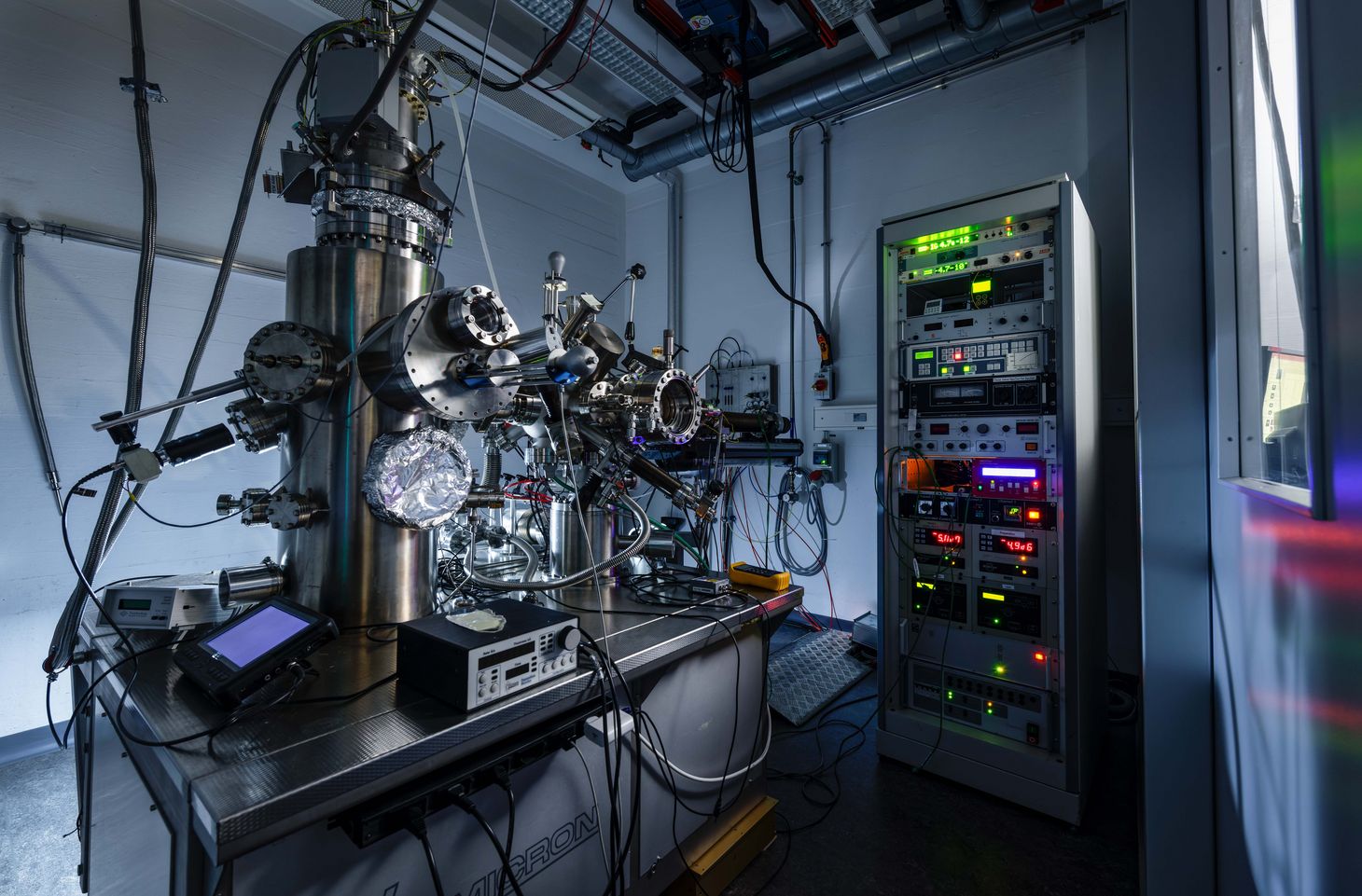