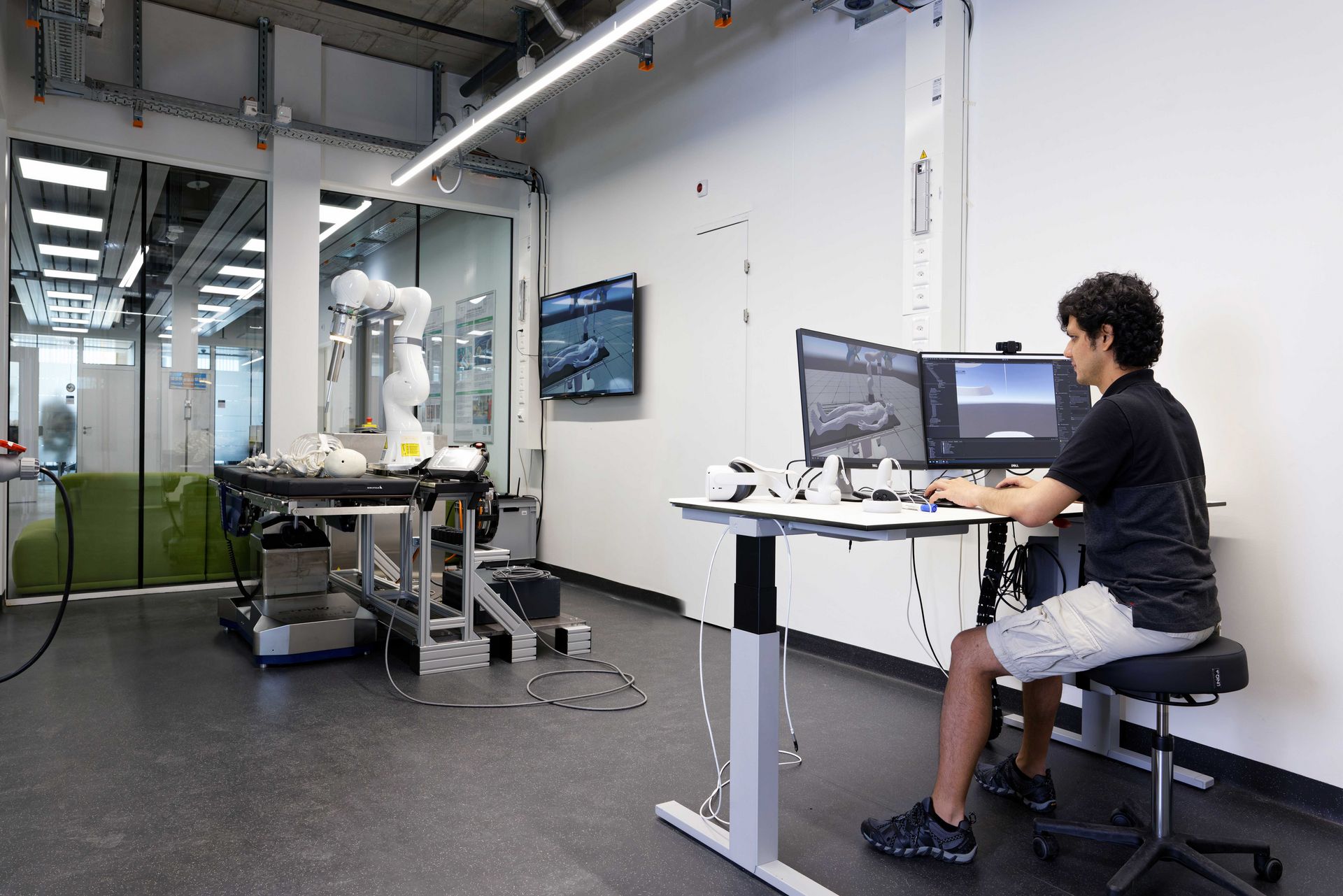
Medical care of tomorrow
Medical interventions are continually becoming more precise, more sophisticated and less invasive. Three projects supported by the Werner Siemens Foundation are driving the developments in medical technology forward—each in its own way.
Getting it just right can be a question of life and death—especially in medical care. The more precisely a tumour can be removed or treated with radiation, the better a patient’s chance of healing. The more effectively a drug can zero in on a pathogen, the lower the risk of side effects. And the higher the resolution of an X-ray or an MRI scan, the greater the likelihood of an early diagnosis.
In medical research, there are various approaches for making medical interventions and diagnostic examinations more accurate. One of the most important and promising of these methods is miniaturisation: because very small instruments have a much less disruptive impact on the body, patients experience fewer traumatic consequences. Nevertheless, building smaller, lighter and more versatile devices requires much more than medical knowledge. That’s why the field of medical technology combines expertise from medical sciences with that from engineering, IT, electrotechnology, chemistry, biotechnology and other disciplines.
Minimally invasive laser scalpel
A prime example of this kind of interdisciplinary collaboration is found at the University of Basel Department of Biomedical Engineering, where the MIRACLE II team are shaping the future of bone surgery. The research groups led by Philippe Cattin, Georg Rauter and Florian Thieringer are creating a robot-guided laser scalpel to enable minimally invasive, high-precision surgical interventions—that are planned and monitored using an innovative virtual and augmented reality system.
“The technology we need for our dream device has yet to be developed,” says Philippe Cattin, professor of image-guided therapy. “That’s why we’re exploring miniaturisation in all areas of our research. We’re trying to push the boundaries of the possible, whether with our robotics platform or in laser optics.” This is critical because the team’s novel surgical robot will only make the leap from lab to clinic if each and every aspect of the project can overcome existing limitations.
Development of the robot itself is the remit of Georg Rauter, professor of surgical robotics. The basic idea is to create an instrument that functions much like a human hand: the “arm” will be positioned in the operating theatre, outside the patient’s body, and surgeons will use it to guide the finger-shaped, jointed appendage at the tip—the endoscopic mini-robot.
Benefitting from Swiss precision
Rauter’s team are seeking to miniaturise both parts of the system. The “arm” is currently quite bulky and takes up too much space in the operating theatre. “Our goal is to have a hand-held system that can be mounted on the operating table,” Rauter says. By contrast, the foremost, finger-like appendage of the robotic system is already incredibly tiny, with a diameter of just eight millimetres. Nevertheless, there are still size-related challenges: currently, the “finger” is made of aluminium but, to be safe for use in the body, it must be built from materials like titanium or stainless steel. “And it’s almost impossible to fabricate something this small with those materials,” Rauter says.
Fortunately, the Swiss watch industry is specialised in the construction of high-precision components made of stainless steel and titanium. Rauter has now asked a mechanical workshop active in the watch industry to manufacture the parts for their robot. He says it may sound simple to have a component built using a different material. “But,” he adds, “in reality it’s a big step.”
In another part of the project, a laser for cutting bone is being integrated into the tiny robot. This task has been assigned to Ferda Canbaz, head of the Center for Intelligent Optics. “Up to now, we’ve been using a bundle of optical fibres with a diameter of roughly three millimetres to supply the tip of the endoscope with enough power,” Ferda Canbaz says. “Now, we’re aiming to channel the laser energy through a single optical fibre.” One of the ideas they’re pursuing is converting the optical fibre itself into a laser medium. “To do this, you shoot a laser at the optical fibre, whereupon the laser light is generated in the optical fibre itself,” Canbaz explains.
Different means, same end
Philippe Cattin’s group has developed two techniques for continually monitoring the endoscope’s location in the body. The first utilises optical interference in the optical fibres: “We interpret the interference and reflected light to determine the bending of the optical fibres—and thus where in the body the robot is located.” The second technique is based on minuscule angle measurement sensors that are just one cubic millimetre; these tiny devices are used to determine the robot’s position by means of the joint angle. Cattin is pleased: “The sensors are a really nice accomplishment. Not only are they much smaller than anything on the market, they’re also up to fifty times more accurate.”
It’s not unusual for researchers to seek several solutions for the same problem. After all, it’s impossible to know in advance whether a concept will work, and whether it can at some point be made marketable. Another example of multiple approaches to solve a single problem are the ideas being developed to prevent the smart laser from cutting into the wrong tissue. Ferda Canbaz and her team are applying a spectroscopic method that uses plasma light to determine what kind of tissue is being cut; at the same time, they’re also investigating an optical system that measures the depth and shape of an incision as well as the temperature at the site of the cut.
Meanwhile, Georg Rauter’s robotics team are tackling the problem from yet another angle. They’ve developed a technology that uses springs instead of a cable drive to connect the joints of the endoscope robot to the motors. “When the endoscope bumps into something, the spring reacts immediately, without the robot knowing about it,” Rauter explains. This means shocks that would otherwise damage tissue in the body are absorbed.

MIRACLE II
Gentle, minimally invasive, robot-guided and highly precise bone surgery—this is the aim of the MIRACLE II project at the University of Basel. The team are developing an endoscopic laser robot capable of making ultra-exact incisions in bones, while miniature sensors and a 3D software program are used to promote patient safety during surgery. In the hospital’s inhouse 3D-printing lab, made-to-measure implants will be fabricated to fit into the pre-cut bones. All of which means that bones can heal faster after an intervention.
Funding from the Werner Siemens Foundation
12 million Swiss francs
Project duration
2022 to 2027
Project leaders
Prof. Dr Philippe Cattin, Department of Biomedical Engineering (DBE), University of Basel
Prof. Dr mult. Florian M. Thieringer, DBE, University of Basel and University Hospital Basel
Prof. Dr Georg Rauter, DBE, University of Basel
> about the project
An implant that heals
As in the MIRACLE II project, bone injuries are the focus of the “Smart implants” project at Saarland University, Saarland University Medical Center, the Center for Mechatronics and Automation Technology and the German Research Center for Artificial Intelligence. The team led by Professor Bergita Ganse and Professor Tim Pohlemann are aiming to develop an implant that not only monitors the healing process of complex fractures but that also promotes healing through its autonomous, activating movements.
To realise their multi-tasking implant, the researchers are integrating a wire made of nickel and titanium—so-called shape-memory alloys, which are materials that can assume two different states, depending on the temperature. The alloys can be moved back and forth, like an artificial muscle, and they’re able to transfer large amounts of energy in a small space.
In a recently published study, the Saarland researchers used simulations to show for the first time how powerful one of the implant’s “micro-massages” should be to support healing. The results showed that, depending on the type of fracture and healing phase, the implant’s range of motion would have to be restricted to between 0.1 and 0.5 millimetres. “In this micro-movement, very large forces are transferred, up to several hundred kilogrammes,” as Tim Pohlemann explains. Indeed, one of the project’s major challenges lies in managing this area of tension between micro-movements and macro-forces.
Data in the soles of their shoes
Another difficulty the team face is determining which patients need a micro-massage—and when. For this, the implant must have access to precise data on stress and strain in the fracture gap. These data are gathered through the sensors in the smart implants themselves, but also from insoles fitted with sensors that patients wear in their shoes. “The conventional approach would be to collect relevant data, analyse the information and then send it all back to the implant,” Bergita Ganse says. “But our vision is to create automated, AI-controlled data interpretation—that way, the implant can function autonomously.”
The researchers have already compiled data in profusion in their specialised gait lab, where the insole-sensors record patient data on strain, acceleration and weight-bearing. “We’ve learned a lot about how these parameters change in patients who are healing well—and also how they change when healing is suboptimal,” Bergita Ganse says. “And we’re now able to use the gait and movement data to assess early on whether or not a fracture will heal well.”
The team have also made progress in another crucial part of the project. “We asked ourselves how we’ll be able to prove that it’s actually the implant that impacts healing,” Ganse says. Their solution is to measure blood flow in the fracture tissue. By doing so, the researchers were able to demonstrate that circulation and oxygen saturation change during a favourable healing process. In future, this knowledge will enable them to measure whether massaging the site of a fracture will—as they believe—improve these parameters.

Immense potential
At present, the smart implant has a long way to go before it’s ready for market entry, as Tim Pohlemann says. Currently, they have a demonstrator, but before they can use it to build a prototype, they must first make several advances. In short, it will take years before a highly complex, auto-regulating implant that’s safe for use in the human body will be approved for medical care. “We have to plan many steps in advance—but also streamline the project wherever possible,” Pohlemann explains.
One potential simplification involves controlling how the implant should move in the body. “At first, we were thinking the implant would need complete freedom to move in all directions,” Tim Pohlemann explains. “But, when dealing with the kinds of forces that are involved in the motions, it’s almost impossible to control mechanically.” To solve the problem, the researchers are exploring variations with a somewhat limited range of movement that are much easier to manufacture. “We probably don’t need to have the device moving in every single direction.”
Tim Pohlemann and Bergita Ganse are convinced that the Saarland smart implant model can do far more than treat bone fractures. “After all,” Pohlemann says, “we virtually look through skin and can influence body processes from the outside. The approach has immense potential.” One possible application would be treating osteoporosis or bone tumours that cause bones to disintegrate over time. In such cases, a minimally invasive implant could increase bone stability while simultaneously serving as a sensor: should the implant detect a decrease in stability, a surgeon can operate to prevent more serious damage.
Regenerating damaged cartilage
The TriggerINK project at DWI – Leibniz Institute for Interactive Materials in Aachen is also focusing on influencing healing processes from outside the body. Researchers in the teams of Laura De Laporte, Stefan Hecht, Andreas Herrmann and Matthias Wessling are developing a novel strategy for regenerating damaged cartilage—in cases of osteoarthritis, for example. Put simply, their vision is to form a supportive hydrogel scaffold made of a gelatinous substance—starting from a bio-ink—into a damaged joint. Once the structure is in place, innovative techniques and materials designed by the researchers will trigger a process of cartilage regeneration along the scaffold.
TriggerINK is emblematic of an important paradigm shift in the field of medicine. “For the past several decades now, there’s been a movement away from conventional surgical interventions towards minimally invasive or even regenerative strategies,” says Laura De Laporte, professor of Macromolecular Materials for Medicine at RWTH Aachen University and member of the DWI scientific board.
Initially, the regenerative approach consisted mainly of cultivating replacement tissue in the lab and then implanting it in the body. “Unfortunately, this procedure often failed because the cultivated tissue integrated poorly into the surrounding body tissue,” De Laporte explains. And when the attempt was made to grow tissue directly inside the body, a serious problem arose: the method utilises proteins and other signals that are instrumental for embryonic development and wound healing—but that also play a role in tumour growth.
“It’s absolutely essential to ensure that these techniques don’t trigger unwanted growth,” De Laporte stresses. That’s why researchers have turned to a new tactic which involves programming materials with functions that can be steered and set in motion. “This is our goal at TriggerINK,” De Laporte says. The researchers work with tools such as magnetic fields, photochemistry, ultrasound and the emerging field of mechanobiology.

Smartimplants
In future, intelligent implants fitted in a bone will be able to directly monitor how well a lower-leg fracture is healing. In addition to stabilising the broken bone, the implants will provide information on the healing progress and detect incorrect weight-bearing. If a bone isn’t healing well, the implant will react and, if necessary, trigger targeted movements to actively stimulate healing at the site of the fracture. The Smart implants project is conducted by a research team at Saarland University Medical Center.
Funding from the Werner Siemens Foundation
8 million euros
Project duration
2019 to 2025
Project leaders
Prof. Dr Tim Pohlemann, Prof. Dr Bergita Ganse, Saarland University Medical Center
> about the project
Molecular switch with visible light
Photochemistry is the domain of Stefan Hecht, Einstein Professor at Humboldt-Universität zu Berlin and associated researcher at DWI Aachen. His team is developing an innovative method to enable the polymer molecules in the bio-ink to link with each other and form a lattice-like gelatinous hydrogel structure. Specifically, they want to form this hydrogel directly in the wound by irradiating the polymer solution. The light stimulates the molecules in the bio-ink so that they begin to crosslink at certain points. Over time, they form the structured scaffold that supports growth of the cartilage tissue.
The crosslinking already works when the bio-ink is exposed to UV rays. “But our aim is to use visible light that’s gentler on the cells,” Stefan Hecht explains. This is easier said than done, as using a different wavelength calls for intense, complex research. “We’ve designed a whole array of different molecules, but the first of these didn’t deliver the desired results.” Recently, however, his team scored a success and proved that the principle of a molecular photoswitch based on visible light is indeed feasible.
They’ve also already identified optimal parameters for the hydrogel—how stiff it should be, for example—to best enable fast tissue regeneration. The researchers are also taking steps to ensure that the tissue grows in the right direction. This is achieved by means of rod-shaped microparticles in the bio-ink that are aligned via a magnetic field. Initial findings have shown that stem cells inside scaffolds with this kind of orientation differentiate into cartilage cells more effectively.
Targeted release of molecules
Nevertheless, even if the hydrogel parameters and alignment are perfect, the stem cells still need some assistance to differentiate and regenerate the tissue. To ensure the cells get the help they need, the researchers are integrating biologically active, cell-growth promoting molecules into the bio-ink. Andreas Herrmann’s group is working on ways to release these molecules by means of external ultrasound signals—that are sent at the right time and right place, deep within the regenerated tissue.
Last year, the researchers used an immunostimulatory molecule in living animals to show for the first time that a local release triggered by ultrasound is possible. In their experiment, they injected the compound into the tail vein of mice and waited until the substance spread via the blood vessels through the entire body. The researchers then activated the molecule via ultrasound—targeting only the liver of the animals, and this with pinpoint precision.
Like their WSS colleagues in the Smart implants project, the TriggerINK team also seek ways to promote healing via external stimulation, although their project functions on an even smaller scale. Instead of using an autonomous implant, the Aachen researchers are working with microgels in combination with gold nano particles. The nanoparticles are activated externally with safe infrared light that penetrates into the printed construct. The pulsing light causes the microgels to contract at regular intervals—every second, for example—and then expand again.
“We’ve taken a big step here, too,” Laura De Laporte says. “Initial tests have shown that the microgels we synthesised and set in motion do indeed activate the cells.” With this achievement, the team have met the first requirement: they’ve demonstrated that stimulation can influence the growth and regeneration properties of cartilage tissue.
It’s fair to say that the three micro-medical projects supported by the Werner Siemens Foundation are well underway. And despite their differences, they all pursue a common goal: improving medical care by making it less invasive, less traumatic and more patient-friendly.

TriggerINK
Regrowing damaged cartilage using a scaffold made of bio-ink: this is the aim of researchers in the TriggerINK project at DWI – Leibniz Institute for Interactive Materials in Aachen. First, a 3D printer injects the bio-ink into a wound, where it is infused with light and aligned via a magnetic field. Then, ultrasound is applied to activate or release the agents and growth factors contained in the bio-ink at set times. If their method works, it promises to revolutionise cartilage regeneration therapies.
Funding from the Werner Siemens Foundation
10 million euros
Project duration
2022 to 2026
Project leaders
Prof. Dr-Ing. Laura De Laporte, DWI – Leibniz Institute for Interactive Materials and RWTH Aachen University
Prof. Dr Stefan Hecht, Humboldt-Universität zu Berlin, associated researcher at DWI Aachen
Prof. Dr Andreas Hermann, DWI and RWTH Aachen University
Prof. Dr Matthias Wessling, DWI and RWTH Aachen University
> about the project
