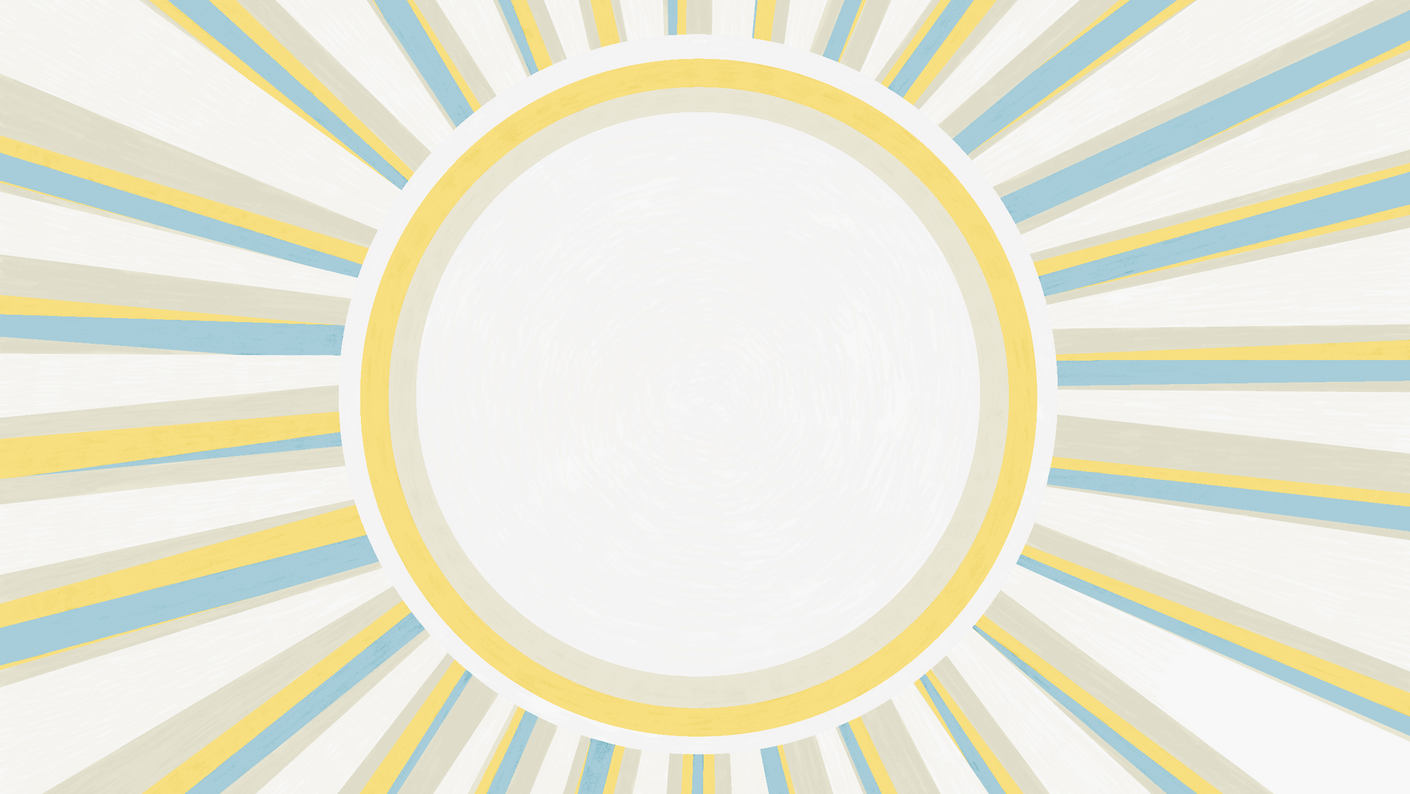
Future energy
To ensure the future of our planet, it’s essential that we reduce our dependency on oil, gas and coal. We must also find new ways to exploit alternative energies—and formulate clear political strategies to drive this massive societal shift forward. Three innovative research projects financed by the Werner Siemens Foundation are seeking solutions.
It’s hardly a big secret: planet Earth is getting hotter and hotter. Today, global temperatures are 1.2 degrees Celsius warmer than 150 years ago, with the trend being even stronger in Central Europe. The root cause is human-made: we release vast quantities of greenhouse gases into the atmosphere—mainly by burning coal, oil and gas to generate energy.
Right now, humanity is at an inflection point: if we fail to stop the warming trend, we’ll soon be facing dire consequences. There will be more—and more intense—heatwaves, forest fires and storms. Ice caps and glaciers will melt, while floods and droughts will render entire regions uninhabitable. What’s needed is an energy transition: a shift away from fossil fuels and towards renewable energies such as solar and hydropower, wind energy, geothermal energy and bioenergy. And time is running out. Signatories to the Paris Climate Accords agreed to keep the rise in mean global temperature to well below 2 degrees Celsius, preferably limiting the increase to 1.5 degrees. To realise the goal, the world’s carbon emissions must decrease by fifty percent by 2030 and reach net zero by 2050.
Achieving a transformation on this scale is incredibly challenging, says Ottmar Edenhofer, co-director and chief economist at the Potsdam Institute for Climate Impact Research (PIK) as well as leader of FutureLab CERES, a project that has received funding from the Werner Siemens Foundation since 2022. “It requires a broad portfolio consisting of various kinds of renewable energies but also strategies and technologies.” Edenhofer divides the challenges into three action areas, the first of which is decarbonising the electricity sector. In future, electricity must stem from renewable energies rather than fossil fuels.
The second is a transformation in transportation and heating systems. “Here, we’re talking about direct and indirect forms of electrification,” Edenhofer says. “Direct electrification” means replacing oil heating with heat pumps powered by electricity, or switching from combustion engines to electric vehicles. By contrast, “indirect electrification” refers to the sustainable production of fuels—“green” hydrogen, for example, or synthetic fuels. The third action area concerns negative emission technologies (NETs) such as capturing and storing CO2 from the atmosphere. Edenhofer says it will be impossible to entirely eliminate greenhouse gas emissions—those emitted in industry or agriculture, for instance—adding, “We’ll have to compensate for them if we want to meet our climate targets.”
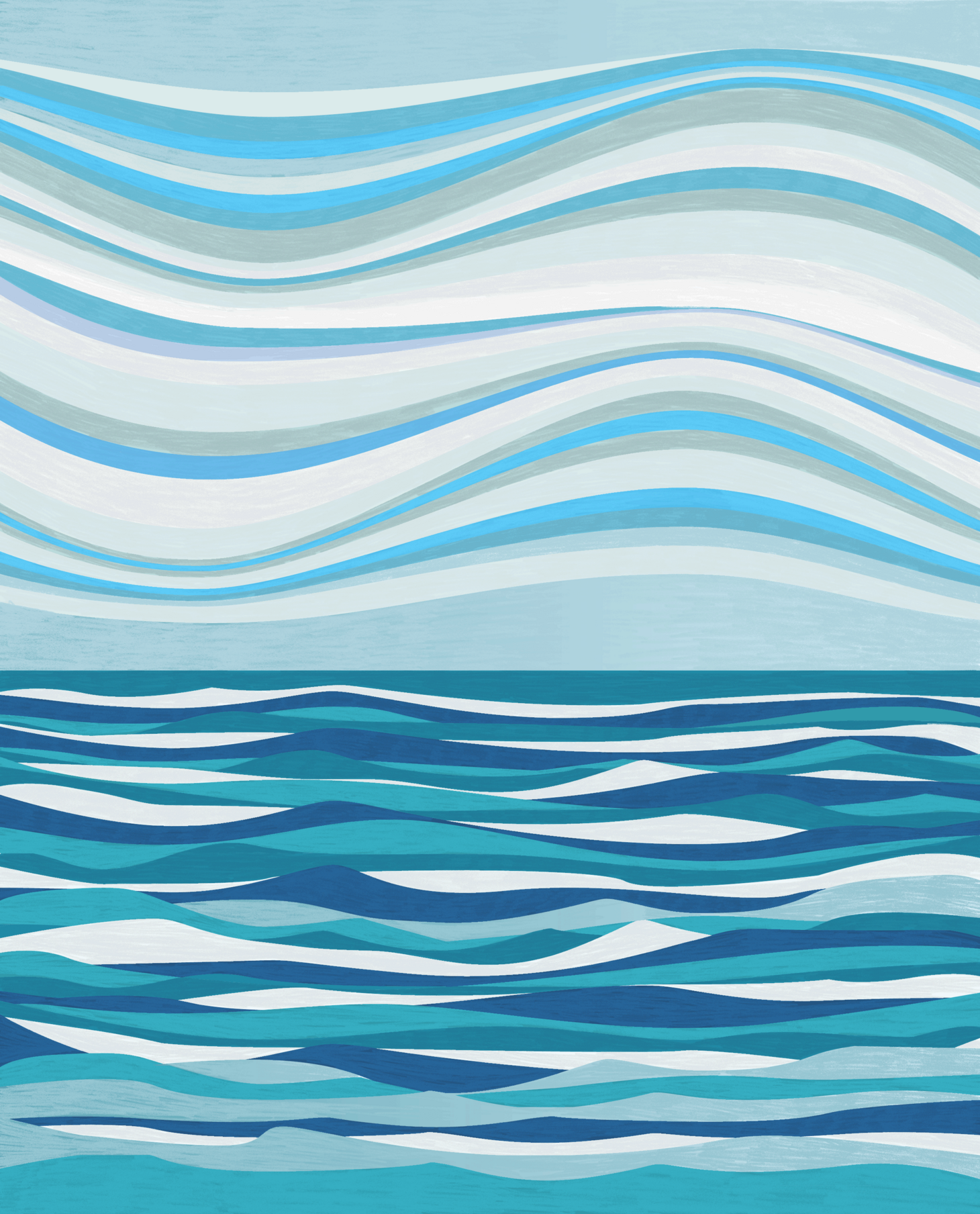
No crystal ball for energy forecasts
Edenhofer further explains that, at present, not all the technologies necessary for the transformation have been developed. “I have to disagree with people who say all we need is a little political will to get the job done.” Carbon storage technologies in particular are by no means available on the scale required. There’s also quite a bit of research to be done on synthetic fuels as well as storage and battery technologies.
And, Edenhofer continues, while all these areas are full of potential, it’s practically impossible to predict which will ultimately prevail. He illustrates this point with an example from the UN Intergovernmental Panel on Climate Change (IPCC), where he acted as co-chair of the Working Group III from 2008 to 2015. “We analysed numerous scenarios describing which technologies would be best for renewable energies,” he says. Only one—compiled by Greenpeace—was based on a major increase in solar energy production. Edenhofer included the peer-reviewed scenario in the final report, a decision that drew quite a bit of ire, as the entire scenario was deemed unrealistic. “And yet,” he says, “when we look at it today, this extreme scenario is the only one that came close to predicting how things have actually developed. All others grossly underestimated photovoltaics.”
This is why he advises caution when policymakers and society go to great lengths to predict technological advances. “Creating conditions that foster competition for the best new technologies is more important.” He would like to see Europe doing more in this area. For instance, he envisions an innovation authority that sets priorities in basic research and organises auctions to drive innovation and help promising technologies achieve a breakthrough.
More electricity—especially in winter
Domenico Giardini, professor of seismology and geodynamics at ETH Zurich, agrees that it’s by no means certain which technologies will be viable in the long term. What is clear, however, is that we’ll be needing much more electricity in the near future. “Just a few years ago, there were politicians who said we could compensate energy consumption by saving energy. Today we know that this doesn’t work.” What’s more, these additional quantities of energy don’t just need to be generated—they must also be distributed and stored.
In particular solar power is much more plentiful in summer than in winter. “However, we consume much more electricity in winter than in summer,” Giardini points out. “This means we need to find ways to generate more energy in winter, but also ways to store summer energy.” Enter geothermal energy. The technology has two main advantages. First, the heat of our planet is a nearly inexhaustible source of potential energy. Five kilometres beneath the surface, the earth’s temperatures range from 160 to 200 degrees Celsius—and that all year round. If we could tap into this heat, we would have a year-round supply of energy.
Second, subterranean rock layers could also be used to capture and store heat, not just release it. “Ten years ago that wasn’t a priority, but today, heat storage is an incred-ibly important aspect in future energy strategies,” Giardini says. Storage capacities of batteries or reservoirs are insufficient for long-term solutions, he adds. “That’s why we’re studying whether we can use underground rock layers as a kind of energy bank: in summer we deposit energy into the rock, and in winter we withdraw it.”
Domenico Giardini heads the BedrettoLab, a unique research facility located below the Saint-Gotthard Massif in Ticino, Switzerland’s southernmost canton. Thanks to funding from the Werner Siemens Foundation, a former ventilation arm in the Furka Tunnel—part of the Matterhorn-Gotthard railway line—was converted into an underground lab for deep geothermal energy. In the meantime, the lab doesn’t only serve ETH Zurich scientists: research and industry partners from all over the world take advantage of the facility as an ideal platform for safely testing deep geothermal energy technologies in near-authentic conditions.
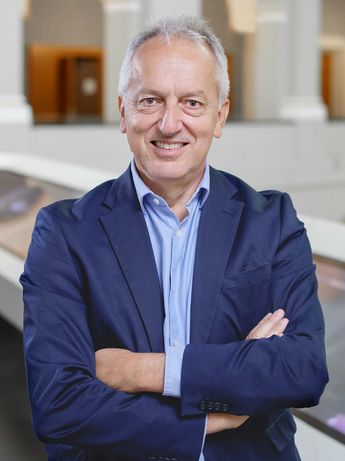
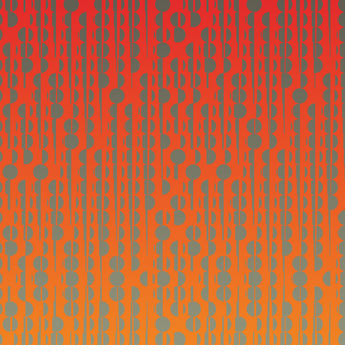
Bedretto Underground Lab
The Werner Siemens Foundation financed the construction of two test beds in the unique underground lab located in the southern Saint-Gotthard Massif, and it continues to fund research projects on deep geothermal energy conducted in the facilities. In the near-authentic conditions of the Bedretto Underground Lab (BedrettoLab), ETH Zurich researchers and their partners from Switzerland and abroad have an ideal environment for studying the physics of earthquakes and for testing methods to safely use and store geothermal energy.
Funding from the Werner Siemens Foundation
12 million Swiss francs
Project duration
2018 to 2024
Project leader
Prof. Dr Domenico Giardini,
professor of seismology and geodynamics, ETH Zurich
New insights into earthquakes
In addition to experiments with new geothermal technologies, researchers are using the facility to better understand how, and under what conditions, earthquakes occur. Safeguarding against earthquakes is always a concern in deep geothermal energy systems, Giardini explains: “Whenever we build underground, we cause the stress levels in the earth’s crust to change, which can trigger earthquakes.” And because the BedrettoLab is located more than a kilometre beneath the earth’s surface, it’s an excellent place to study and measure how rock layers change when exposed to such pressure.
To set up the experiments, the researchers descended even deeper than the lab itself: they drilled several boreholes of up to 400 metres beneath the facility’s ground floor, then affixed hundreds of sensors inside the boreholes to measure changes in temperature and stress as well as displacements in the rock. When carrying out their experiments, the researchers inject water into the borehole, where the sensors provide detailed information on how this kind of stimulation triggers microquakes. “Up to now, we had a very unclear picture of what happens. We thought the earthquakes were made up of a kind of cluster of small tremors,” Giardini explains. “But the data we collected in the immediate vicinity of the microquakes showed that there are really large faults of eighty to one hundred metres in length. They move in the form of small tremors—it’s very craggy rock.” Thanks to the findings, the team can now make better models of what happens when water is injected into rock layers.
Gaining more information about these rock movements is also important for the latest endeavour in the BedrettoLab: in collaboration with Azienda Elettrica Ticinese, the energy supplier in Ticino, and with funding from the Swiss Federal Office of Energy, the researchers launched a new project to study seasonal energy storage. The basic idea is to use energy generated from photovoltaics and other sustainable sources for heating water and storing it in underground rock layers.
“It’s a demonstration project,” Giardini explains. “Next summer we’ll start injecting hot water—heated to up to eighty degrees—into the rock and studying the effects.” Key questions concern what happens when water is injected and then extracted. Does it cause tremors? If yes, can the tremors be controlled by injecting water in shorter intervals or by being more careful when injecting the water? Does the hot water have a long-term impact on the rock layers? Then, in the following winter, the researchers aim to find out how much energy can be removed from the reservoir and used for heating.
The team in the BedrettoLab now face the challenge of building a new side tunnel, a project funded by the European Research Council (ERC). Construction began in the autumn of 2023 and will take another three years to complete. Progress is slow, as rock blasting operations have to be conducted repeatedly—and, of course, there are also experiments to be conducted. Because the new side arm will run parallel to a large fault in the rock, the researchers can use it as the starting point for drilling more boreholes up to the fault. They’ll then install more sensors in these boreholes for measuring seismic activity. “This will deliver yet more information about what happens in a fault when the rock starts moving—and about how tremors start and stop,” Giardini says.
Carbon dioxide and hydrogen storage
It’s fair to say that the two technologies of geothermal energy generation and the underground storage of surplus energy—but also carbon dioxide and hydrogen—generate great interest. Now, to transport the earth’s heat to the surface, Martin O. Saar, Werner Siemens Foundation Endowed Chair, and his geothermal energy and geofluids research group at ETH Zurich are exploring potential options other than using hot water (the current method) as a heat conduit. He’s even come up with an idea that would create two solutions in one: a method he helped develop known as CO2 Plume Geothermal (CPG), which combines deep geothermal energy generation and carbon storage—killing two birds with one stone, as it were.
When the greenhouse gas CO2 is stored between 2.5 and 3 kilometres below ground, it naturally heats up to at least 100 degrees Celsius. With his CPG method, Saar wants to exploit this heat by creating a circuit: the hot carbon dioxide is brought to the surface, where it’s used directly to drive turbines. After it cools off, the gas is channelled back into the underground storage facility. As such, the CO2 injected at the start is permanently stored. And, as Saar explains, because carbon dioxide is less viscous than water, and because it expands much more when heated, it achieves a higher heat production rate—which generally more than compensates for the lower heat capacity of CO2 compared to water. “It’s also a cost-effective way of exploiting rock layers that have both low permeability and low temperatures. And when heated enough, CO2 rises up to the surface almost on its own.” Overall, Saar continues, CPG has the potential to double or triple the energy output for direct heating or power generation.
After investing more than twelve years into researching and developing the method, Saar recently founded the CPG Consortium, a collaboration between industry and academia in which currently two large mineral oil companies are paying members. The aim is to study the method in a large-scale pilot project and then commercialise the technology.
Another of Saar’s future goals is the underground injection and conversion of hydrogen. “Although the idea is simple, putting it into practice is very difficult,” he says. The concept is to combine carbon dioxide and sustainably produced “green” hydrogen with specific kinds of microorganisms in the subsurface. The tiny organisms utilise the earth’s heat to transform the hydrogen and carbon dioxide molecules into methane. If needed, the methane can be retrieved at the surface and used to generate energy. “Methane is well suited and quite safe as an energy storage medium,” Saar says. “The big advantage compared to the original hydrogen molecules is that it can be distributed and used via already existing natural gas infrastructures—and that this methane would be carbon neutral.
Like Domenico Giardini, Martin O. Saar, too, is convinced that the energy transition will only succeed if we use a combination of different kinds of available energy and methods. “We need solutions that can be optimised according to region,” he says, and adds that studies have demonstrated an energy mix is always more cost-effective than systems that depend on one, or a few, kinds of energy. For example: in order to supply a region with solar and wind energy at any time of the day or year, large overcapacities must be generated.
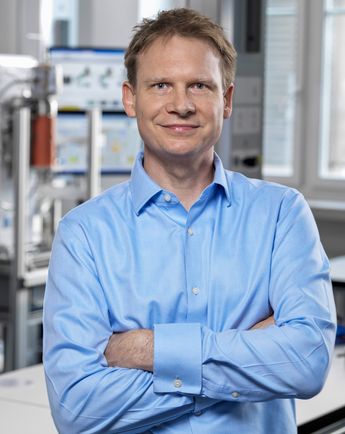
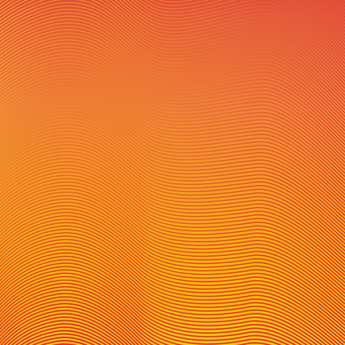
Deep geothermal energy
The earth’s heat is one of the largest unused energy reserves on the planet. At ETH Zurich, Martin O. Saar and his team are exploring ways to harness this heat for large-scale energy production. In one area of research, Saar teamed up with industry partners to develop a new drilling technology—and an innovative method that can permanently sink carbon dioxide into the earth while also using it to generate geothermal electricity.
Funding from the Werner Siemens Foundation
10 million Swiss francs
Project duration
2015 to 2024
Project leader
Prof. Dr Martin O. Saar,
professor of geothermal energy and geofluids at ETH Zurich
Generating reserve energy
Saar is also convinced that deep geothermal energy could play a key role in the energy mix of the future. It’s capable of supplying both base load and reserve energy, depending on demand. And reserve energy supplies are related to yet another area he’s involved in. With funding from the Swiss Innovation Agency Innosuisse, he’s leading a consortium of research and industry partners in a project to study how a method called Advanced Geothermal Systems (AGS) could contribute to realising the energy transition in Switzerland—and throughout the world.
The idea is to build power plants that function much like the heat pumps used in residential buildings: at a depth of five to ten kilometres below the earth’s surface, two boreholes are, in simplified terms, joined together to form a U-shaped loop. Inside the loop, heat is extracted from the rock using carbon dioxide as a circulation fluid. The heat is then either used directly or converted into electricity in a power plant and fed into the grid. If employed across the whole country, the method would be an ideal replacement for the unecological gas-fired power plants that currently supply reserve energy, especially as the closed loop enables the system to be started up and shut down again very quickly.
At present, however, the method is too expensive, mainly due to the high cost of drilling, as Saar explains. This aspect has motivated the project consortium to further develop a drilling method known as Plasma Pulse Geo Drilling (PPGD), which works with a type of electric shock rather than mechanically breaking up the rock. The innovative technology requires only about a quarter of the energy consumed in standard drilling procedures, and the electrodes needed for the electric pulses last much longer than standard drill bits.
Much work remains until this type of ecological power plant is ready for the market. On the bright side, however, Saar says lab experiments suggest that the new drilling method will work well at great depths. “Although lab tests demonstrate that the higher temperatures in the earth—the kind we want to access—seem to slow the drilling process a little, the high rock pressures at those low levels are very advantageous,” Saar explains. “All in all, it looks like drilling with PPGD will most likely be easier the deeper we penetrate.”
CERES off to a good start
Innovative technologies are naturally essential for the energy transition. However, it’s also clear that the shift to a new, sustainable energy future will be expensive—and that society as a whole must commit to change. Indeed, it’s essential that new technologies and solutions also find a consensus among policymakers and in society. At FutureLab CERES, Ottmar Edenhofer and his research team want to find out which innovative technologies and which policy instruments can best promote the sustainable management of natural resources. And last year, the project made excellent progress. For example, in June, the FutureLab team attended a large conference in Cyprus, where they presented a detailed overview of their entire project. “Our presentation met with a great deal of interest in the research community,” Edenhofer says.
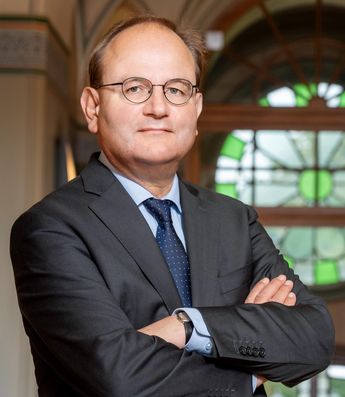
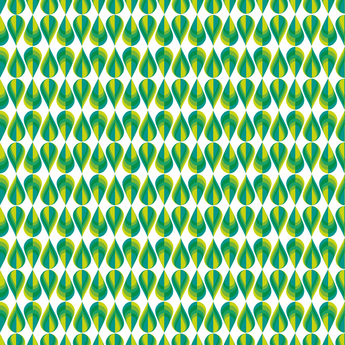
FutureLab CERES
The team at FutureLab CERES at the Potsdam Institute for Climate Impact Research (PIK) are seeking to understand which policy instruments will best promote the sustainable management of natural resources. The spotlight is placed on countries like Brazil, Indonesia and the Democratic Republic of the Congo, where biodiversity is exceptional, where climate change is a particularly large threat—and where huge revenues are generated through the extraction of fossil fuels and other natural resources.
Funding from the Werner Siemens Foundation
10 million euros
Project duration
2022 to 2031
Project leader
Prof. Dr Ottmar Edenhofer,
co-director and chief economist at the Potsdam Institute for Climate Impact Research (PIK), Potsdam bei Berlin
To learn more, the researchers have begun examining the dynamics of the coal phase-out in the Global South. One aspect of particular significance for climate policy is how countries like Brazil, Colombia, Vietnam or South Africa can replace coal-fired power plants—or what it is that prevents them from taking this step. Edenhofer explains that phasing out coal is a massive undertaking for these countries—for both economic and political reasons. “This process isn’t only about the power plants themselves. Turbine manufacturers, auxiliary steam systems, power generators and engineering companies are also affected.” In other words, the entire supply chain for production and distribution is already standing, and it’s trimmed for fossil fuel power plants.
To address these issues, researchers at PIK and the Mercator Research Institute on Global Commons and Climate Change (MCC) in Berlin have begun studying aspects such as the role of public credit institutions in the construction of new coal-fired power plants. They discovered that cross-border loans, chiefly from China, Japan and South Korea, are the main drivers for new coal-fired power plants in the Global South. And that these credits are often a means to an end—namely, they benefit exporters of turbine manufacturers and other suppliers in the lending countries.
CERES has also made excellent progress in a second area of research concerning policy measures. Governments often enact whole sets of such measures: bans, taxes, subsidies and dialogue. The Potsdam researchers want to understand which factors distinguish impactful energy policy and sustainability measures—and what makes them less effective. “Previously, our data came from Europe,” Edenhofer says, “but now we’ve analysed policy measures in almost every country on the planet, and then interpreted these data using machine learning.” Although the results aren’t yet published, Edenhofer says they basically show that, despite having many differences, effective measures share considerable commonalities. The researchers now plan to draw on the findings to formulate new ideas and proposals—and drive the energy transition forward.
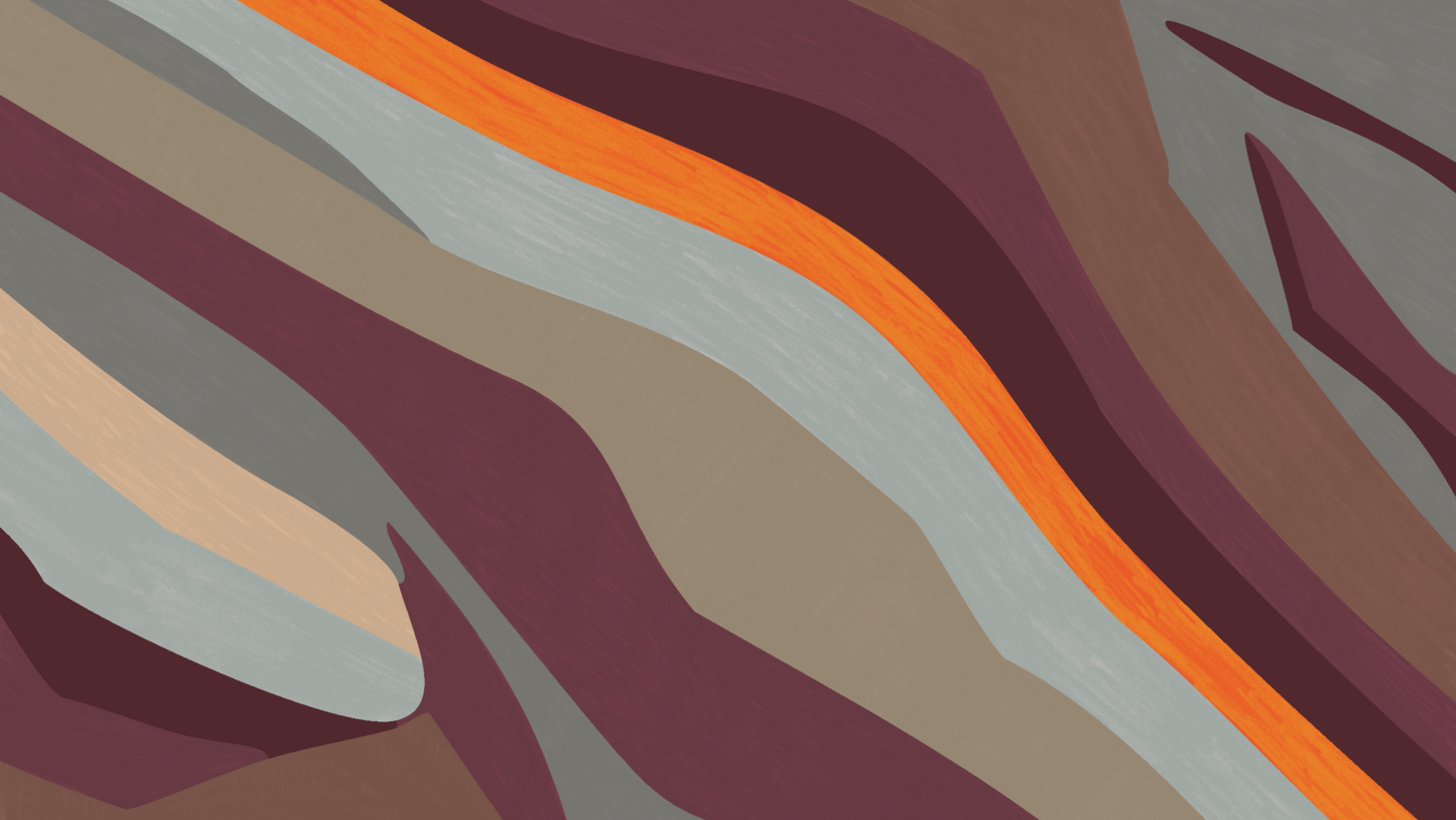